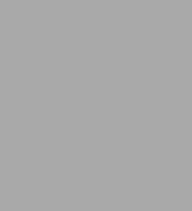
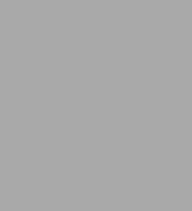
Paperback
-
PICK UP IN STORECheck Availability at Nearby Stores
Available within 2 business hours
Related collections and offers
Overview
The world of quantum physics is generally thought of as hopelessly esoteric. While classical physics gives us the laws governing why a ball rolls downhill, how a plane is able to fly, and so on, its quantum cousin gives us particles that are actually waves, "spooky" action at a distance, and Schrodinger's unlucky cat. But, believe it or not, even the most mundane of everyday activities is profoundly influenced by the abstract and exotic world of the quantum.
In Breakfast with Einstein, Chad Orzel illuminates the strange phenomena lurking just beneath the surface of our ordinary lives by digging into the surprisingly complicated physics involved in his (and anyone's) morning routine. Orzel, author of How to Teach Quantum Physics to Your Dog, explores how quantum connects with everyday reality, and offers engaging, layperson-level explanations of the mind-bending ideas central to modern physics.
From the sun, alarm clocks, and the red glow of a toaster's hot filaments (the glow that launched quantum mechanics) to the chemistry of food aroma, a typical day is rich with examples of quantum weirdness. Breakfast with Einstein reveals the hidden physics all around us, and after reading this book, your ordinary mornings will never seem quite as ordinary again.
Product Details
ISBN-13: | 9781946885357 |
---|---|
Publisher: | BenBella Books, Inc. |
Publication date: | 12/11/2018 |
Pages: | 272 |
Sales rank: | 358,627 |
Product dimensions: | 6.00(w) x 8.90(h) x 0.80(d) |
About the Author
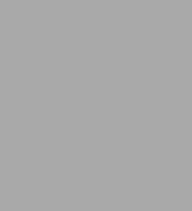
Read an Excerpt
CHAPTER 1
SUNRISE: THE FUNDAMENTAL INTERACTIONS
The sun comes up not long before my alarm clock starts beeping, and I get out of bed to start the day ...
It might seem like cheating to start a book on the quantum physics of everyday objects by talking about the sun. After all, the sun is a vast sphere of hot plasma, a bit more than a million times the volume of Earth, floating in space ninety-three million miles from here. It's not an everyday object in the same way as, say, an alarm clock that you can pick up and throw across the room when it wakes you after a too-short sleep.
On the other hand, in a sense the sun is the most important everyday object of all, even beyond the glib observation that a day doesn't start until the sun rises. Without the light we receive from the sun, life on Earth would be utterly impossible — the plants we rely on for food and oxygen wouldn't grow, the oceans would freeze, and so forth. We're dependent on the light and heat of the sun for our entire existence.
For the purposes of this book, the sun is also a useful vehicle for a kind of dramatis personae, introducing the key players of quantum physics: the twelve fundamental particles that make up ordinary matter, and the four fundamental interactions between them.
The twelve fundamental particles — particles that cannot be broken down any further into even smaller parts — are divided into two "families," each with six particles. The quark family consists of the up, down, strange, charm, top, and bottom quarks, and the lepton family contains the electron, muon, and tau particles, along with electron, muon, and tau neutrinos. The four fundamental interactions are gravity, electromagnetism, the strong nuclear interaction, and the weak nuclear interaction. You can often find these particles and interactions enumerated on a colorful chart hanging in a physics classroom, collectively referred to by the sadly generic name "the Standard Model of physics." The Standard Model encapsulates everything we know about quantum physics (and also about the ability of physicists to come up with catchy names), and ranks as one of the greatest intellectual achievements of human civilization. The sun is a perfect introduction to the Standard Model, because all four of the fundamental interactions have a role to play in order for the sun to shine.
So, we'll start our story with the sun, taking a whirlwind tour of its inner workings to illustrate the essential physics that powers everything else we do. We'll go through each of the fundamental interactions in turn, beginning with the best known and most obvious of these forces: gravity.
GRAVITY
If you were to generate sports radio–style "power rankings" of the fundamental interactions of the Standard Model, three of the four have a decent case for claiming the top spot. If pressed to make a choice, though, I'd probably give the honors to gravity, because gravity is ultimately responsible for the existence of stars, and thus for most of the atoms making up our bodies and everything around us, enabling our silly conversations about ranking fundamental forces.
In our everyday lives, gravity is probably the most familiar and inescapable of the fundamental interactions. It's gravity that you fight against when getting out of bed in the morning, and gravity that keeps me from being able to dunk a basketball (well, gravity, and being woefully out of shape ...). We spend the vast majority of our lives feeling the pull of gravity, which makes its temporary absence — as in amusement park rides featuring sudden drops — fascinating, and even thrilling.
That familiarity also makes gravity one of the most-studied forces in the history of science. People have been thinking about how and why objects fall to the earth for at least as long as we have records of people pondering the workings of the natural world at all. Popular legend traces the origin of physics to a young Isaac Newton being struck (literally, in some versions) by the fall of an apple from a tree, and thus impelled to invent a theory of gravity. Contrary to the image conjured up by this apocryphal tale, though, scientists and philosophers were already well aware of gravity, and had devoted significant thought to how it works. By Newton's day, experiments by Galileo Galilei, Simon Stevin, and others had even made some quantitative headway on the subject, establishing that all dropped objects, regardless of their weight, fall toward the earth with the same acceleration.
As an old man, Newton himself recounted a version of his apple encounter to younger colleagues. There's no mention of it in materials from around when it would've happened (while he was working on gravity), but he did spend an extended time during that period at his family's farm in Lincolnshire, when the universities were closed due to an outbreak of plague. To the extent that there's truth to the story, however, the most popular telling misidentifies the nature of Newton's insight. Newton's epiphany was not about the existence of gravity but its scope: he realized that the force pulling an apple to the ground is the same force that holds the moon in orbit about the earth, and the earth in orbit around the sun. In the Philosophiae Naturalis Principia Mathematica, Newton proposed a universal law of gravitation, giving mathematical form to the attractive force between any two objects in the universe having mass. This form, combined with his laws of motion, allowed physicists to explain the elliptical orbits of the planets in the solar system, the constant acceleration of objects falling near the earth, and a host of other phenomena. It established a template for physics as a mathematical science, one that is followed right up to the modern day.
The crucial feature of Newton's law of gravity is that the force between masses depends on the inverse of the distance between them squared — that is, if you halve the distance between two objects, you get four times the force. Objects that are closer together experience a stronger pull, which explains why the inner planets of the solar system orbit more rapidly. It also means that a diffuse collection of objects will tend to be drawn together, and as they grow closer, they are compressed ever more tightly by the increasing force of gravity.
This increasing force is critical for the continued existence of the sun, and it's the ultimate source of its light. The sun is not a solid object, but rather a vast collection of hot gas, held together only by the mutual gravitational attraction of all the individual atoms making it up. While it may top our list in terms of everyday impact, gravity is the weakest of the fundamental interactions by a mind-boggling amount — the gravitational force between a proton and an electron is a mere 0.000000000000000000000000000000000000001 times the electromagnetic force that holds them together in an atom. The enormous quantities of matter present in the sun, however — some 2,000,000,000,000,000,000,000,000,000,000 kg — build up a gigantic collective gravitational force, pulling everything nearby inward.
A star like the sun begins life as a small region of slightly higher density in a cloud of interstellar gas (mostly hydrogen) and dust. The extra mass in that region pulls in more gas, increasing its mass, and thus increasing the gravitational attraction to pull in more gas still. And, as new gas falls in toward the growing star, it begins to heat up.
At the microscopic scale, a single atom drawn toward a protostar speeds up as it falls inward, just like a rock dropping toward the surface of the earth. You could, in theory, describe the behavior of the gas in terms of the speed and direction of each of the individual atoms, but that's ridiculously impractical even for objects vastly smaller than a sun-sized ball of gas — not just because of the number of atoms, but because the atoms interact with each other. A non-interacting atom would be drawn in toward the center of the gas cloud, speeding up as it went, then would pass out through the other side, slow down, stop, and turn around to repeat the process. Real atoms, though, don't follow such smooth paths: they hit other atoms along the way. After a collision, the colliding atoms are redirected, and some of the energy gained by the falling atom as it accelerated due to gravity is transferred to the atom it hit.
For a large collection of interacting atoms, then, it makes much more sense to describe the cloud in terms of the collective property known as temperature. Temperature is a measure of the average kinetic energy of a material as a result of the random motion of the components making it up — for a gas, this is mostly a function of the speed of the atoms zipping around. An individual atom is pulled inward and accelerates, gaining energy from gravity and increasing the total energy of the gas. When it collides with other atoms, that energy is redistributed, raising the temperature. The total energy doesn't increase, but rather than having a single fast-moving atom passing through a bunch of slower ones, after many collisions, the average speed of every atom in the sample increases by a tiny amount.
The increasing speed of the atoms in the cloud of gas tends to push it outward, as a faster-moving atom can travel a greater distance from the center before gravity turns it around and brings it back in. The redistribution of energy from new atoms, though, means that this increase isn't enough to stop the collapse, and as new atoms are pulled in, the mass of the protostar increases, increasing the gravitational force. This, in turn, draws in more gas, bringing in more energy and more mass, and so on. The cloud continues to increase in both temperature and mass, becoming denser and denser, and hotter and hotter.
Left unchecked, the growing force of gravity would crush everything down to an infinitesimal point, forming not a star but a black hole. While these are fascinating objects, warping space and time and presenting a major challenge to our most fundamental theories of physics, the environment near a black hole is not a hospitable place to have a weekday morning breakfast.
Happily, the other fundamental interactions have their own roles to play, halting the star's collapse and allowing the formation of the sun we know and love. The next to kick in is the second most familiar: electromagnetism.
ELECTROMAGNETISM
We regularly encounter the electromagnetic interaction in everyday life, whether in the form of static electricity crackling in a load of socks fresh from the dryer, or that of magnets holding gradeschool artwork to the refrigerator. Unlike gravity, which is always attractive, electromagnetism can be either attractive or repulsive — electric charges come in both positive and negative varieties, and magnets have both north and south poles. Opposite charges or poles attract each other, while like poles or charges repel. The electromagnetic interaction is even more ubiquitous than static charges and magnets, though — in fact, it's responsible for our ability to see, well, anything.
In the early 1800s, electromagnetism was a hot topic in physics, with many phenomena involving electric currents and magnets being studied for the first time. Among those studying electromagnetism was British physicist Michael Faraday. He is responsible for a number of technical advances that play key roles in an everyday morning, including his work on liquefying gases, which finds application in refrigeration, and the development of the "Faraday cage" that (among many other things) helps contain the electromagnetic fields used to cook your food to the inside of your microwave oven. Unquestionably, his most important discovery was that not only can electric currents affect nearby magnets, but moving magnets and changing magnetic fields can create current — which is the basis of the vast majority of commercial electricity generation powering the conveniences of modern life. He was one of the first to understand the behavior of charges and magnets in terms of electric and magnetic fields filling empty space and telling distant particles how to move.
Faraday is a seminal figure in physics, one of three people whose likenesses Einstein displayed in his office (the other two were Newton and James Clerk Maxwell). Alas, he came from a poor background, and while he was a great experimenter with deep physical insight, he lacked the formal mathematical training needed to translate this insight into a form that would convince the physicists of his day to take the "field" concept seriously. It fell to James Clerk Maxwell, from a well-off Scottish family, to put electric and magnetic fields on a firm mathematical foundation. In the 1860s, Maxwell showed that all known electric and magnetic phenomena could be explained by a simple set of mathematical relationships — in modern notation, there are four "Maxwell's equations," compact enough to fit on a T-shirt or coffee mug. Faraday's electric and magnetic fields are real things, connected to each other in deep ways — a changing electric field will create a magnetic field, and vice versa.
Maxwell's equations encompass all known electric and magnetic phenomena, and also predicted a new, unified one: electromagnetic waves. If an oscillating electric field is combined in the right way with an oscillating magnetic field, the two will support one another as they travel through space, the changing electric field causing a change in the magnetic field, which causes a change in the electric field, and so on. These electromagnetic waves travel at the speed of light, and light was already known to behave like a wave; Maxwell's equations were quickly embraced as an explanation for the nature of light — namely, that it is fundamentally an electromagnetic phenomenon. Electromagnetism provides the basis for understanding how light and matter interact, and as we'll see in chapters to come, probing the nature of the interactions between material objects and electromagnetic waves sets the stage for many of the discoveries that established quantum mechanics.
Electromagnetic forces are also largely responsible for the familiar structure of the objects we encounter each day. Ordinary matter is made up of atoms, which are themselves made up of smaller particles distinguished by their electric charge: positively charged protons, negatively charged electrons, and electrically neutral neutrons. An atom consists of a positively charged nucleus containing protons and neutrons, surrounded by a cloud of electrons drawn in by the electromagnetic attraction of the nucleus.
As mentioned earlier, the electromagnetic interaction is vastly stronger than gravity — a fact nicely demonstrated by the party trick of rubbing a latex balloon on your hair and then sticking it to the ceiling. In the rubbing process, a tiny fraction of a percent of the atoms in the balloon steal an electron from atoms in your hair, giving the balloon a small negative charge. The attraction between this tiny charge and the atoms in the ceiling is strong enough to hold the balloon in place, resisting the gravitational pull of the entire Earth, with a billion billion billion times the mass of the balloon.
The strength of electromagnetism is an indispensable factor in producing the sun. Electromagnetic interactions are responsible for the collisions between atoms that convert the energy gained from gravity into heat. As the temperature of the gas falling into a growing star increases, it eventually becomes hot enough — around 100,000 kelvin or almost 180,000 degrees Fahrenheit — to separate the electrons in hydrogen atoms from the protons in the nucleus, producing a gas of electrically charged particles: a plasma. Gravity continues to compress the plasma, but the mutual repulsion between the positively charged protons holds them apart, resisting gravity's pull. As the forming star continues to draw in more gas, the temperature increases to higher and higher levels.
Despite the enormous disparity between electromagnetism and gravity, though, the plasma can't escape gravity entirely because the electrons that were part of the gas cloud are still around. They're moving too fast to be captured by protons to make atoms, but they keep the star as a whole electrically neutral. If protons alone were present, the mutual repulsion of such an enormous collection of positive charges would blow the whole star apart in an instant. Thanks to the neutralizing background of electrons, though, any individual proton feels only the force of its few nearest neighbors, while the gravitational pull compressing the star comes from the mass of every single particle. As more gas is added, the gravitational force gets stronger and stronger, eventually overwhelming the electromagnetic force.
Electromagnetic interactions can slow the compression of a hot plasma collapsing under gravity, but electromagnetism alone can't stop the collapse and produce a stable star. To create the stable sun as we know it requires an enormous release of energy leading to even higher temperatures, which brings us to the next player in our story: the strong nuclear interaction.
(Continues…)
Excerpted from "Breakfast with Einstein"
by .
Copyright © 2018 Chad Orzel.
Excerpted by permission of BenBella Books, Inc..
All rights reserved. No part of this excerpt may be reproduced or reprinted without permission in writing from the publisher.
Excerpts are provided by Dial-A-Book Inc. solely for the personal use of visitors to this web site.
Table of Contents
IntroductionChapter 1 Sunrise: The Fundamental InteractionsChapter 2 The Heating Element: Planck’s Desperate TrickChapter 3 Digital Photos: The Patent Clerk’s HeuristicChapter 4 The Alarm Clock: The Football Player’s AtomChapter 5 The Internet: A Solution in Search of a ProblemChapter 6 The Sense of Smell: Chemistry by ExclusionChapter 7 Solid Objects: The Energy of UncertaintyChapter 8 Computer Chips: The Internet Is for Schrödinger’s CatsChapter 9 Magnets: How the H*ck Do They Work?Chapter 10 Smoke Detector: Mr. Gamow’s EscapeChapter 11 Encryption: A Final Brilliant MistakeConclusionAcknowledgmentsIndexWhat People are Saying About This
“Using his morning routine as an example, Orzel sets out to show the reader how quantum physics is a part of our everyday lives, and he largely succeeds in this informative and friendly book . . . The conceit of following Orzel around for his morning routine offers the barest of frames, but he uses it to great effect.”—New York Times Book Review
"Physics is everywhere and in every thing, and no one explains physics better than Chad Orzel. This book is a meal for your mind."
—John Scalzi
"Fans of quantum physics will be informed and entertained."
—Booklist
"Common wisdom paints quantum mechanics as one of the most abstract and esoteric of subjects, daunting for non-experts. Yet, as Chad Orzel wonderfully shows in Breakfast with Einstein, a full gamut of our commonplace daily activities—from boiling water for tea on a glowing range to taking and exchanging photos with our electronic cameras and phones—depends on quantum rules. By focusing on how quantum mechanisms guide the workings of his typical morning routine, Orzel cleverly brings those important principles close to home.”
—Paul Halpern, author of The Quantum Labyrinth: How Richard Feynman and John Wheeler Revolutionized Time and Reality
"Prof. Orzel draws us in with the everyday experience. And then we find we are on a journey of more than 100 years of physics. The reader is rewarded not only with a deeper understanding of everyday things, but also learns how physicists themselves look the world every day."
—David Saltzberg, professor of physics and astronomy at UCLA
“Why don’t light bulbs fry us with deadly radiation? Why can’t you stick your hand through a solid wall? Why isn’t every scrap of metal a magnet? So many science books focus on the latest wacky cosmic discovery, but Orzel shows how the ordinary world around us is already plenty weird.”
—George Musser, contributing editor at Scientific American and author of Spooky Action at a Distance
“William Blake saw the world in a grain of sand. Chad Orzel sees the universe in a slice of toast. Orzel is a master at bringing abstract ideas like relativity and quantum mechanics down to earth without ever skimping on the science. This fun, engaging, and deeply informative book is definitive proof that everything is fascinating when you look closely enough. I’ll never see my breakfast the same way again.”
—Amanda Gefter, author of Trespassing on Einstein’s Lawn
“Breakfast with Einstein offers a clear and entertaining introduction to the wonders of quantum mechanics, showing that these principles surround us and are employed regularly in our everyday lives. Chad Orzel is the perfect guide to the world of atoms and photons, demonstrating that even our morning breakfast rituals are not possible without the wonders of modern physics.”
—James Kakalios, professor of physics at the University of Minnesota and author of The Physics of Superheroes and The Physics of Everyday Things
“Chad Orzel's new book is a masterfully told story about the myriad ways that physics shapes our lives.”
—Sabine Hossenfelder, physicist and author of Lost in Math