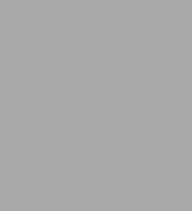
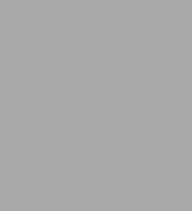
eBook
Available on Compatible NOOK devices, the free NOOK App and in My Digital Library.
Related collections and offers
Overview
At the tips of our trowels, a sun-driven world of microbes, insects, roots, and stems awaits—and it is a world no one knows better than James Nardi. A charming guide to all things green and growing, Nardi is as at home in prairies, forests, and wetlands as he is in the vegetable patch. And with Discoveries in the Garden, he shows us that these spaces aren’t as different as we might think, that nature flourishes in our backyards, schoolyards, and even indoors. To find it, we’ve only got to get down into the dirt.
Leading us through the garden gate, Nardi reveals the extraordinary daily lives and life cycles of a quick-growing, widely available, and very accommodating group of study subjects: garden plants. Through close observations and simple experiments we all can replicate at home, we learn the hidden stories behind how these plants grow, flower, set seeds, and produce fruits, as well as the vital role dead and decomposing plants play in nourishing the soil. From pollinators to parasites, plant calisthenics to the wisdom of weeds, Nardi’s tale also introduces us to our fellow animal and microbial gardeners, the community of creatures both macro- and microscopic with whom we share our raised beds. Featuring a copse of original, informative illustrations that are as lush as the garden plants themselves, Discoveries in the Garden is an enlightening romp through the natural history, science, beauty, and wonder of these essential green places.
Product Details
ISBN-13: | 9780226531830 |
---|---|
Publisher: | University of Chicago Press |
Publication date: | 12/22/2022 |
Sold by: | Barnes & Noble |
Format: | eBook |
Pages: | 289 |
Sales rank: | 776,431 |
File size: | 9 MB |
About the Author
Read an Excerpt
CHAPTER 1
Seeds
I have great faith in a seed. Convince me that you have a seed there, and I am prepared to expect wonders.
Henry David Thoreau
A seed is a marvel of creation; from so simple a beginning and with only energy from the sun and nutrients from air and soil, each seed transforms into a complete plant with leaves, stems, roots, flowers, fruits, and seeds of its own. As you can see if you open a seed such as a bean seed, a miniature version — the embryo — of the future plant is preformed in this small parcel of just a few cells. These cells of the seed are destined to form all future plant parts; through their many divisions during the plant's life, the basic form of each plant contained in the seed grows and matures. What is also remarkable about the transformation of a seed to a plant is that this basic form of the plant is retained throughout all the growth that takes place. Growth is never haphazard and disorganized but orchestrated by groups of cells at root tips and stem tips, where growth and cell division are concentrated. As the plant grows, these cells will continue to divide to produce more cells like themselves but also cells that become specialized leaf cells, root cells, flower cells. The dividing cells remain in specific places throughout the plant called buds and meristems. Each seed carries these untold promises and possibilities. A Welsh proverb well expresses what wonders can unfold when a seed sets forth on its journey of germination and growth: "A seed hidden in the heart of an apple is an orchard invisible."
The Future Plant Is Preformed in Its Seed
OBSERVE: The most conspicuous features of a germinating bean seed or sunflower seed are the seed leaves, or cotyledons (cotyle = cup-shaped); these provide the first nutrients for the baby plant or embryo and are the first part of the future plant to disappear as the nutrients they contain are transferred to the growing seedling. Each germinating seedling sends one growing tip into the soil below the cotyledons — the future root or hypocotyl (hypo = below; cotyle = cotyledon) — and the other growing tip into the sky above the cotyledons, the future stem, or epicotyl (epi = above; cotyle = cotyledon). Split almost any seed that you have soaked in water for several hours along its plane of least resistance, and you will reveal the future plant or embryo hidden within (fig. 1.2).
Seeds of flowering plants begin their sheltered lives within flowers that then transform into fruits as the seeds mature. All flowering plants, or angiosperms (angio = enclosed; sperm = seed) form fruits with enclosed seeds that have either one cotyledon or two cotyledons — no more, no less. The cotyledons of these seeds are an important feature that distinguishes the two major lineages of the flowering plants. Of the 235,000 species of angiosperms, 65,000 species have seeds with one cotyledon; these plants include corn, wheat, oats and all grasses, asparagus, onions, lilies, irises, palms, and orchids. These are referred to as monocots (mono = one; cot = abbreviation for cotyledon). Melons, beans, tomatoes, cabbage, and carrots are among the remaining 170,000 species whose seeds have two cotyledons; these are the dicots (di = two; cot = abbreviation for cotyledon).
Evergreen conifers — pines, spruces, firs — also have seeds with epicotyls, hypocotyls, and cotyledons that are surrounded by nutritive tissue. Their seeds, however, do not lie within protective fruits, but instead lie exposed on the surfaces of their cone scales. For this reason they are referred to as gymnosperms (gymnos = naked; sperm = seed). All living gymnosperms — conifers and their other relatives with naked seeds — make up only 720 species worldwide, representing a mere 0.3 percent of all plants with seeds. By producing both seeds and spores, angiosperms and gymnosperms are distinguished from other green plants such as mosses, ferns, and algae that produce only spores and have never acquired the ability to form seeds. (Additional information on seeds and spores is provided in the section of chapter 4 titled "The Difference between Seeds and Spores.")
Like the seeds of many flowering plants, the seeds of many conifers — pine nuts — are relished for their flavor and nutrition. An inside view of a pine nut reveals its resemblance to flavorful seeds of angiosperms like the peanut (fig. 1.3). The pine embryo and its cotyledons, however, are embedded in maternal nutritive tissue found only in gymnosperms.
Peanuts are relatives of beans; and whenever you munch on peanuts, you are munching mostly on cotyledons of peanut embryos. Within each peanut, the future peanut plant is nestled between the two large cotyledons at one end of the seed. Look closely and you can make out the peanut embryo's future leaves pointing inward and its future root pointing outward.
In the teardrop-shaped apple seed, the future root lies at the tapered end of the seed and points outward. On top of this future root lies a tiny group of cells destined to become the first leaves and the trunk of the apple tree. The cotyledons occupy the broad end of the apple seed and meet at the point where the future root joins the future shoot.
As they first form inside fruits, all angiosperm seeds begin life endowed with a store of nutrients in the form of a tissue called endosperm (endo = within; sperm = seed). Endosperm tissue arises at the time of pollination, at the same time that the angiosperm embryo is conceived. Unlike the maternal nutritive tissue that surrounds the pine embryo, the endosperm is both maternal and paternal in origin. Chapter 4describes the details of how the pollination of a flower with a single pollen grain can produce not only an embryo but also its accompanying endosperm. Embryonic plants found in seeds (such as those of apples, beans, sunflowers, and peanuts) with conspicuous cotyledons transfer most of the stored nutrients from their endosperms to their cotyledons long before they germinate.
The angiosperm seeds we have examined so far have done just that; by the time they germinate, their endosperms have completely disappeared. Many seeds, however — such as tomatoes, corn, peppers, rye, and wheat — do not have such conspicuous cotyledons. These seeds have retained their original endosperms as a reservoir of nutrients that they can tap for nourishment as they germinate. Views of the interiors of a rye seed, a pepper seed, and a corn seed show these differences in endosperms and cotyledons, plus the first days of germination for different seeds (fig. 1.4).
HYPOTHESIZE: What happens if you gently remove the two cotyledons from a newly germinated bean seed? Compare the growth of a bean seedling having both of its cotyledons with a bean seedling that has lost both or one of its cotyledons. Can whatever nutrients that are derived from cotyledons ever be replaced by nutrients from other sources? Can beans that have lost one or both cotyledons ever grow as large as beans with both cotyledons? When can cotyledons be removed from bean seedlings without having any influence on the development of the future bean plant?
How does a newly sprouted sunflower respond after five or six hours if one or both of its cotyledons are gently wrapped with foil to block light from reaching the cells of the cotyledons? What happens if the epicotyls of these newly sprouted seedlings are removed with fine scissors and only their cotyledons remain? The observed results should be less puzzling after reading about the experiments of Charles and Francis Darwin in chapter 2.
Knowing Which Way to Sprout
OBSERVE: Knowing the difference between up and down is important to all plants. In each seed we saw that the future leaves point in one direction and the future root points in the opposite direction. The future roots and future leaves have definite orientations within seeds. When seeds are carefully planted or even scattered in the garden, however, they can be placed in any position and any orientation and still manage to send their roots down and their leaves up. Every seed has a distinct sense of direction. A kernel of corn is one of the best seeds in which to demonstrate this ability of plants to distinguish up from down; the first root of corn emerges from the pointed end of the seed and the first leaf emerges from the flat end. The large seeds of corn are easy to glue on dry filter paper in different orientations (fig. 1.5). As you are sitting at your desk or table, glue some pointing toward you, some pointing to your left, some pointing to your right, and some pointing away from you. Once the glue has thoroughly dried, moisten the filter paper and cover the dish to hold the moisture for a few days. Also tape the lid to the 100-millimeter petri dish so it remains in place when you now move the dish from its horizontal position to a vertical position that germinating seeds would naturally occupy in the soil. Do the first roots from the four corn seeds all grow in the same direction?
HYPOTHESIZE: Let the first roots grow about one inch. What do you think will happen to these growing roots of corn if you rotate the petri dish 180 degrees? What happens if you place the dish in a horizontal position? In this position the roots are prevented from growing downward; they can only grow up or grow to the side.
Making sure the filter paper is moist, flip the horizontal dish upside down. The filter paper and seeds should remain on the original bottom of the petri dish; the roots and shoots of the corn seedlings now must adjust to being upside down, being able to grow downward and sideways but not upward.
How are roots able to sense the difference between up and down? Just as our own sense of up and down is conveyed by the movement of tiny granules that rub against tiny cell structures within a chamber of our inner ear, the cells of a plant's growing root tip contain small, round, dense granules called statoliths (stato = resting; lith = stone) that shift about in the cell as the cell changes position (fig. 1.6). These statoliths turn out to be dense starch granules that form in special chlorophyll-free chloroplasts called amyloplasts (amylo = starch; plast = form). Figure I.6 in the introduction represents a good example of this transformation from chloroplast to amyloplast. When the statoliths settle to the bottom of the cells, the root grows downward and growth occurs uniformly around the root tip. But if the root is placed on its side, the statoliths settle on that side of the cell. Now more growth occurs on the opposite side of the root, and the root starts growing down. What happens when the root tip is placed upside down and the statoliths settle to the tops of the cells? Somehow this shift in the statoliths prompts the root tip to do an about-face and reverse the direction of its growth completely. We now understand that the repositioning of statoliths is translated by cells of root tips into directional root growth. The details of the events that transpire between the initial movement of statoliths and the final movement of root tips, however, are still being filled in by carefully planned hypotheses and experiments, many of which are discussed on future pages.
HYPOTHESIZE: If the movement of statoliths within the cells of the root cap conveys information to the root about its orientation in the soil, then one would hypothesize that removal or damaging of the root cap cells would alter the normal response of a root to gravity.
Repeat the previous experiment with corn seeds that have been attached to filter paper in four different orientations. After the first roots have sprouted from the corn seeds, carefully remove their root caps with fine tweezers or a fine pin. Observe the subsequent growth of these roots. How does absence of a root cap and its statoliths influence the direction in which a root grows?
Knowing When to Sprout
A seed remains at rest until it receives the signals from its environment to go into action. These signals tell the seed that conditions are right to begin growing. There is no point in sprouting if the air and soil are too cold or too dry or if you are buried too deep in the dark soil to reach the far-off light.
OBSERVE: To watch the chain of events as a seed germinates and a new plant is born, place radish seeds on the moist surface of damp filter paper placed in the bottom of a covered petri dish. Which part of the future plant emerges first from the seed? What is the fuzzy material that surrounds this first sprout? A close-up view reveals that the fuzz is not mold but represents thousands of hairs that project from the cells on the surface layer of the sprout (fig. 1.7). As this first sprout from the radish seed extends through the soil, its thousands of hairs also extend in all directions throughout the soil, into tiny pores and spaces in search of the water and nutrients essential for its growth. They manage to reach out into the soil without getting hopelessly intertwined and tangled with one another — a feat as remarkable as the ability of the thousands of fish in a school to swim and the hundreds of birds in a flock to fly in their orderly arrays.
Seeds that have been buried deep underground can remain dormant but healthy for many years. Seeds of a wildflower from Siberia currently hold the remarkable record of existing 32,000 years in a dormant but healthy state. Russian scientists reported in 2012 that they had discovered seeds of a flower in the pink family (Silene) that had been stashed away in a ground squirrel's burrow 32,000 years earlier — a time when woolly mammoths shared the landscape with these flowers. Soon after its construction, the burrow had been buried under 125 feet of sediment, and the seeds remained frozen for all those years. Buried seeds face two dilemmas: lack of light energy and lack of oxygen; and they germinate only when they are brought to the surface of the soil.
HYPOTHESIZE: Do different seeds have different environmental requirements for germination? Can all — or only some — seeds germinate in the dark? Seeds that have been placed in dishes with moist filter paper do not encounter a shortage of oxygen; but these seeds may require not only moisture and oxygen but also light before they send forth their first shoot. To test the responses of seeds to the absence and presence of light, place tobacco seeds in three 100-millimeter petri dishes with moist filter paper. After adding a dusting of tiny tobacco seeds to each dish, place one dish in the light and a second dish in complete darkness for five days. Place the third dish in complete darkness for two days; expose it to light for one day; and then return it to the dark for two more days. Follow the same procedure with radish seeds. For some seeds, the presence of moisture and oxygen alone may be sufficient to trigger their germination. Can you think why different seeds may differ in their germination requirements?
Can seeds germinate at any time of year? What about seeds of desert plants that face many months of drought? Many seeds that mature at the end of summer, such as those of apples and oaks and prairie flowers, have to experience the cold days of winter before they will germinate. Rather than germinating at the beginning of winter and soon exposing themselves to freezing temperatures, seeds remain dormant and will not germinate until after they have been exposed to several weeks of temperatures below 40 degrees Fahrenheit. Seeds can experience these temperatures either during a natural winter outdoors or during an artificial winter in a refrigerator. After the passage of winter or a minimum number of days below 40 degrees Fahrenheit (5 degrees Celsius), germination of a seed can proceed without fear of its new sprouts being frost bitten.
While germination for some seeds requires exposure to a critical cold period, certain other seeds must await exposure to fire, to smoke, or simply to rain before they begin sprouting. Seeds from many tropical plants germinate only within a relatively high temperature range of 95 to 104 degrees Fahrenheit (35 to 40 degrees Celsius). Thick, hard, and impermeable seed coats are often cracked by brief exposure to fire, increasing exposure of the seed's inner embryo to moisture and air. While not necessarily essential for germination, smoke exposure of seeds from some prairie plants increases their germination by 30 to 40 percent, as researchers at Chicago Botanic Garden have demonstrated. Seeds use cues for their germination that are molded by the moisture, light, and temperature conditions that seeds must face in their particular habitats.
(Continues…)
Excerpted from "Discoveries in the Garden"
by .
Copyright © 2018 James Nardi.
Excerpted by permission of The University of Chicago Press.
All rights reserved. No part of this excerpt may be reproduced or reprinted without permission in writing from the publisher.
Excerpts are provided by Dial-A-Book Inc. solely for the personal use of visitors to this web site.
Table of Contents
PrefaceIntroduction: Conversing with Plants
1 Seeds
2 Buds and Stems, Stem Cells and Meristems: Growing Up, Down, and Out
3 The Underground World: Bulbs, Tubers, and Roots
4 The Journey from Flower to Fruit and Seed
5 Energy from the Sun and Nutrients from the Soil
6 Movements of Vines and Tendrils, Leaves and Flowers
7 Wisdom of the Weeds: Lessons in How Plants Face Adversity
8 Plant Colors
9 Plant Odors and Oils
10 Fellow Gardeners: Other Creatures Who Share Our Gardens
Epilogue
Appendix A. Important Chemicals in the Lives of Plants
Appendix B. List of Plants Mentioned in the Text
Glossary
Further Reading
Index