Dynamics of Fluids in Porous Media
This classic work by one of the world's foremost hydrologists presents a topic encountered in the many fields of science and engineering where flow through porous media plays a fundamental role. It is the standard work in the field, designed primarily for advanced undergraduate and graduate students of ground water hydrology, soil mechanics, soil physics, drainage and irrigation engineering, and petroleum and chemical engineering. It is highly recommended as well for scientists and engineers already working in these fields.
Throughout this generously illustrated, richly detailed study, which includes a valuable section of exercises and answers, the emphasis is on understanding the phenomena occurring in porous media and on their macroscopic description. The book's chapter titles reveal its comprehensive coverage: Introduction, Fluids and Porous Matrix Properties, Pressures and Piezometric Head, The Fundamental Fluid Transport Equations in Porous Media, The Equation of Motion of a Homogeneous Fluid, Continuity and Conservation Equations for a Homogeneous Fluid, Solving Boundary and Initial Value Problems, Unconfined Flow and the Dupuit Approximation, Flow of Immiscible Fluids, Hydrodynamic Dispersion, and Models and Analogs.
"Systematic and comprehensive . . . a book that satisfies the highest standards of excellence. . . . Will undoubtedly become the standard reference in this field." — R. Allen Freeze, IBM Thomas J. Watson Research Center, Water Resources Research.
1000279756
Throughout this generously illustrated, richly detailed study, which includes a valuable section of exercises and answers, the emphasis is on understanding the phenomena occurring in porous media and on their macroscopic description. The book's chapter titles reveal its comprehensive coverage: Introduction, Fluids and Porous Matrix Properties, Pressures and Piezometric Head, The Fundamental Fluid Transport Equations in Porous Media, The Equation of Motion of a Homogeneous Fluid, Continuity and Conservation Equations for a Homogeneous Fluid, Solving Boundary and Initial Value Problems, Unconfined Flow and the Dupuit Approximation, Flow of Immiscible Fluids, Hydrodynamic Dispersion, and Models and Analogs.
"Systematic and comprehensive . . . a book that satisfies the highest standards of excellence. . . . Will undoubtedly become the standard reference in this field." — R. Allen Freeze, IBM Thomas J. Watson Research Center, Water Resources Research.
Dynamics of Fluids in Porous Media
This classic work by one of the world's foremost hydrologists presents a topic encountered in the many fields of science and engineering where flow through porous media plays a fundamental role. It is the standard work in the field, designed primarily for advanced undergraduate and graduate students of ground water hydrology, soil mechanics, soil physics, drainage and irrigation engineering, and petroleum and chemical engineering. It is highly recommended as well for scientists and engineers already working in these fields.
Throughout this generously illustrated, richly detailed study, which includes a valuable section of exercises and answers, the emphasis is on understanding the phenomena occurring in porous media and on their macroscopic description. The book's chapter titles reveal its comprehensive coverage: Introduction, Fluids and Porous Matrix Properties, Pressures and Piezometric Head, The Fundamental Fluid Transport Equations in Porous Media, The Equation of Motion of a Homogeneous Fluid, Continuity and Conservation Equations for a Homogeneous Fluid, Solving Boundary and Initial Value Problems, Unconfined Flow and the Dupuit Approximation, Flow of Immiscible Fluids, Hydrodynamic Dispersion, and Models and Analogs.
"Systematic and comprehensive . . . a book that satisfies the highest standards of excellence. . . . Will undoubtedly become the standard reference in this field." — R. Allen Freeze, IBM Thomas J. Watson Research Center, Water Resources Research.
Throughout this generously illustrated, richly detailed study, which includes a valuable section of exercises and answers, the emphasis is on understanding the phenomena occurring in porous media and on their macroscopic description. The book's chapter titles reveal its comprehensive coverage: Introduction, Fluids and Porous Matrix Properties, Pressures and Piezometric Head, The Fundamental Fluid Transport Equations in Porous Media, The Equation of Motion of a Homogeneous Fluid, Continuity and Conservation Equations for a Homogeneous Fluid, Solving Boundary and Initial Value Problems, Unconfined Flow and the Dupuit Approximation, Flow of Immiscible Fluids, Hydrodynamic Dispersion, and Models and Analogs.
"Systematic and comprehensive . . . a book that satisfies the highest standards of excellence. . . . Will undoubtedly become the standard reference in this field." — R. Allen Freeze, IBM Thomas J. Watson Research Center, Water Resources Research.
34.95
In Stock
5
1
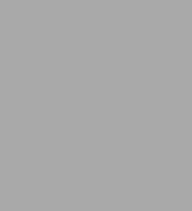
Dynamics of Fluids in Porous Media
800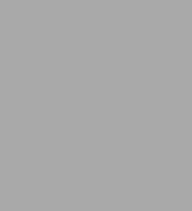
Dynamics of Fluids in Porous Media
800Paperback(Revised ed.)
$34.95
34.95
In Stock
Product Details
ISBN-13: | 9780486656755 |
---|---|
Publisher: | Dover Publications |
Publication date: | 09/01/1988 |
Series: | Dover Civil and Mechanical Engineering |
Edition description: | Revised ed. |
Pages: | 800 |
Product dimensions: | 6.14(w) x 9.21(h) x (d) |
From the B&N Reads Blog