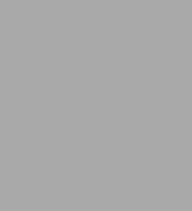
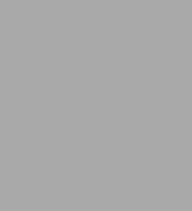
Hardcover
-
PICK UP IN STORECheck Availability at Nearby Stores
Available within 2 business hours
Related collections and offers
Overview
About the Author
Read an Excerpt
Enantioselective Homogeneous Supported Catalysis
By Radovan ?ebesta
The Royal Society of Chemistry
Copyright © 2012 Royal Society of ChemistryAll rights reserved.
ISBN: 978-1-84973-176-8
CHAPTER 1
Ionically-tagged Transition Metal Catalysts
1.1 Introduction
Ionic liquids are liquids consisting solely of ions. Those with melting points below 100 °C are often called room temperature ionic liquids but within this chapter simply the term 'ionic liquids' will be used. Their interesting physical/ chemical properties stimulated the investigation of a great number of research and even technical applications. Their use in synthesis has been summarized in an excellent two-volume book edited by Wasserscheid and Welton.
The concept of ionically-tagged transition metal catalysts develops on the interface of homogeneous and heterogeneous catalysis. It aims to combine positive features of both worlds. The primary function of ionic tags is to enable catalyst recycling. On the other hand, appropriate reaction media offer the possibility of maintaining catalysis in the liquid phase and so benefit from high catalytic activities of homogeneous catalysts. Furthermore, the effect of ionic tags often goes beyond a simple anchor for immobilization and recycling of catalyst. Many reactions are enhanced as a result of an ionic tag installed into the catalyst structure compared to the unmodified catalyst. This effect led Lombardo and Trombini to postulate a concept of electrosteric activation. This means the stabilization of a transition state of a catalytic reaction by electrostatic and steric interaction with ionic tags.
Suitable reaction media for ionically-tagged catalysts can be ionic liquids, supercritical liquids, water or combinations of these. Use of biphasic set-ups is also attractive. This chapter gives an overview of enantioselective catalysis with transition metal based catalysts having an ionic moiety. Ionic catalysts used in water are, however, excluded as they are extensively covered in Chapter 7.
In this chapter, 1-butyl-3-methylimidazolium and 1-ethyl-3-methylimida-zolium cations are denoted as [bmim] and [emim]; 1-butyl-2,3-dimethylimi-dazolium and 1-hexyl-2,3-dimethylimidazolium as [bdmim] and [hdmim]; 1-butylpyridinium cation is denoted as [bpyr]; N-ethyl-3-methylpiccolinium cation is denoted as [epic]. The bis(trifluoromethyl sulfonyl)imide anion is denoted as NTf2, and triflate and tosylate as OTf and OTs.
1.2 Achiral Ionically-tagged Transition Metal Complexes
Highly polar ligands are well known in the coordination chemistry of transition metals. Such complexes were, at first, only used in water. Several reviews and books give a good overview of synthesis and applications of these hydrophilic ligands. However, it was only in 1996 when Chauvin and co-workers suggested that ligands bearing ionic moieties can be used for immobilization of metal complexes in ionic liquids. They noticed that rhodium-catalyzed hydrogenation of pent-1-ene was five times faster in [bmim]PF6 than in acetone. Cationic rhodium complexes were well retained in ionic liquids, which could be reused. However, in rhodium-catalyzed hydroformylation, catalyst leaching was observed. After extraction of organic products, the ionic liquid was reused but part of the rhodium complex was extracted to the organic phase too. Use of mono-and trisulfonated triphenylphosphines as ligands to rhodium completely prevented catalyst leaching to the organic phase.
The concept was then further developed in a number of ways. Various cationic phosphine ligands were tested in rhodium-catalyzed hydrogenations and hydroformylations in ionic liquids or biphasic conditions. Several, structurally different, cations were successfully applied (Figure 1.1). Wasserscheid and Olivier-Bourbigou showed that guanidinium ion is particularly suitable for immobilization of rhodium complexes. van Leeuwen and co-workers attached two imidazolium moieties to Xantphos skeleton. In this way they created ligand 3, which showed excellent recycling results and very little leaching.
Ruthenium-arene catalysts were modi?ed by appending imidazolium moiety as well (Scheme 1.1). Imidazolium ionic tags are designed by their similarity with the most common type of ionic liquids. Therefore, it is also the most frequent type of ionic tag. However, it has also a disadvantage, because the proton in position 2 is acidic; therefore, an imidazolium ion with an alkyl group substituted position 2 is often used.
Palladium-catalyzed Suzuki and Stille couplings as well as Heck reaction were successfully performed with various ionically-tagged catalysts (Figure 1.2). These catalysts have good catalytic activities and at the same time significantly reduced metal leaching from ionic liquid.
The usefulness of the ionic-tagging strategy for catalyst immobilization was also amply demonstrated in ruthenium-catalyzed olefin metathesis. Both Grubbs catalyst of the first and second generation (8–13) have been modified with ionic tags. Usually imidazolium ions have been selected. Figure 1.3 shows various ways in which ionic tags can be introduced into metathesis catalysts.
Achiral Mn-Schiff base 14 was used as an epoxidation catalyst, or porphyrin 15 derivatized with four pyridinium moieties was used for the oxidation of styrene (Figure 1.4).
1.3 Chiral Transition Metal Catalysts with Ionic Tag
Chiral transition metal catalysts can be immobilized through ionic forces in ionic liquids. This is enabled by charge carried by a metal ion. Numerous examples of this approach have been described. Such systems, however, often suffer from considerable metal leaching during work-up operations. The introduction of an auxiliary ionic moiety into the ligand has been suggested as a possible solution to this problem. The installation of an ionic tag into the ligand structure, indeed, helps prevent metal leaching. Catalytic activities are usually comparable or better than that of unmodified catalysts.
1.3.1 Catalysts for Enantioselective Reduction
Enantioselective hydrogenations are one of the most widely employed asymmetric transformations. Expensive rhodium, ruthenium and iridium complexes are the most active hydrogenation catalysts. This motivated great effort for the synthesis of immobilized catalysts. Also, the concept of ionically-tagged catalysts has been developed and tested on hydrogenation reactions. Several researchers therefore tried to immobilize rhodium and ruthenium complexes with the help of ionic tags. An important factor is also good solubility of hydrogen ionic liquids. However, prototypical hydrogenation catalysts, Rh-BINAP complexes, are too air-sensitive and therefore have not been used in ionic liquids. Rhodium complexes with DIOP or DUPHOS have been successfully used in ionic liquids for hydrogenation of functionalized olefins.
The first rhodium catalyst with an ionic tag was prepared by Lee and coworkers. They attached two imidazolium moieties onto a rhodium-disphosphine complex. The resulting catalyst 16 showed excellent catalytic activities in asymmetric hydrogenation of enamides. In the two-phase system [bmim]SbF6/i-PrOH, enamide was hydrogenated with 97% ee with full conversion within 1 hour. The catalyst 16 was recycled four times with very little loss of catalytic activity (95% ee, 85% conversion in 1 h). In comparison, hydrogenation with unmodified complex 17 resulted in a similarly enantioselective reaction in the first run, but in subsequent runs enantioselectivity and conversion decreased considerably (4th run, 88% ee, 85% conversion in 12 h). After the first run the i-PrOH layer was investigated by inductively coupled plasma atomic emission spectroscopy (ICPAES). In experiment with complex 16 no rhodium (<1 ppm) or phosphorus (<3 ppm) leaching from the ionic liquid was detected, whereas with complex 17 it was found that 2% rhodium and 6% phosphorus was leached from the ionic liquid. A control experiment with low catalyst loading (0.5 mol% of complex 17) showed that rhodium leaching is not the sole reason for decreased catalytic performance of the catalyst. Improved stability of the catalyst 16 in ionic liquid was suggested as a possible explanation of its superior catalytic activity (Scheme 1.2).
Ferrocenyl diphosphine ligands serve in a number of excellent hydrogenation systems, including industrial applications. Solvias and Novartis researchers tested ferrocenyl diphosphines, such as Taniaphos, Josiphos, Walphos or Mandyphos, in rhodium-catalyzed hydrogenation in ionic liquids as well as mixtures of ionic liquid with organic solvents or water. The most promising results were obtained in mixtures of ionic liquids with water – wet ionic liquid. For instance, in a mixture of [omim]BF4/H2O ligands Taniaphos and Josiphos afforded enantioselectivities of 99% ee (Scheme 1.3). Josiphos was reused six times with constant ee and only in the seventh run did the reaction time have to be prolonged from 20 to 40 min to reach full conversion. Enantioselectivity, however, remained unchanged (99% ee). Inductively coupled plasma mass spectrometry (ICP-MS) found only 0.9 ppm Rh in the water phase, so the decrease in activity was attributed to catalyst decomposition, rather than to leaching of the catalyst.
Inspired by these promising results, they also synthesized modified Josiphos ligands with imidazolium tag 20 and 21. These diphosphanes were successfully employed in rhodium-catalyzed asymmetric hydrogenation of methyl acetamidoacrylate (MAA) and dimethyl itaconate (DMI) (Scheme 1.4).
Modified ligands 20 and 21 paralleled catalytic behaviour of unmodified ligands in the asymmetric hydrogenation in classical organic solvents as well as under biphasic conditions. Hydrogenation of MAA using ligand 21 proceeded with 99% ee, and for reduction of DMI ligand 20 was superior (99% ee). Imidazolium tagged ligands showed much better reusability in t-BuOMe/ [bmim]BF4 biphasic system compared with unmodified Josiphos ligands (approx. 15% decrease of TOF for the 8th cycle, with constant enantiomeric purity of the product).
Ruthenium-catalyzed transfer hydrogenation of ketones is an important alternative to classical hydrogenation methods. Several attempts were made to prepare reusable ruthenium catalysts with ionic tags. Geldbach and Dyson succeeded in the introduction of the imidazolium moiety onto η6-arene of the Ru complex. Catalyst 22 was slightly less active than unmodified catalyst 23 in the absence of ionic liquid. More importantly, enantioselectivity was significantly lower (58% ee) compared to p-cymene ligand 23 (98% ee). On the other hand, with catalyst 22, dissolved in 1-butyl-2,3-dimethylimidazolium hexafluorophosphate [bdmim]PF6, hydrogenation of acetophenone in a biphasic system (ionic liquid and propan-2-ol/KOH) proceeded well (Scheme 1.5). Using ionic catalyst 22, enantioselectivity was as high as with traditional p-cymene ligand 23 (98% ee). Recycling of the catalytic system led to decreased conversion (52% in the 4th run compared with 99% in the 1st run) of the reaction, but enantioselectivity remained the same. Decrease in catalytic activity was explained by partial leaching of the catalyst from ionic liquid due to decomposition of the ruthenium complex. As an alternative hydride donor to propan-2-ol/KOH, formic acid/triethylamine azeotrop was also used. Enantioselectivities were even higher (99% ee) but again a decrease in conversion between recycling experiments was observed.
A different approach to functionalization of Ru-TsDPEN catalyst 25 was chosen by Ohta and co-workers. They introduced the imidazolium moiety into the diamine part of the ruthenium complex. Using the catalyst 24, transfer hydrogenation of acetophenone with formic acid/triethylamine in [bmim]PF6 proceeded with high enantioselectivity (92% ee) and high conversion (98%). Unmodified Ru-TsDPEN catalyst 25 gave similar results (93% ee, 96% conversion) but after the 4th cycle conversion decreased gradually (4th cycle, 88%; 5th cycle, 63%). In comparison, catalyst 24 showed slightly better results (4th cycle, 92%; 5th cycle, 75%) (Figure 1.5).
Ru-BINAP complexes are important alternatives to their rhodium counterparts. They can be efficiently used for hydrogenation of β-keto esters in ionic liquids. Recycling of Ru-BINAP catalyst in ionic liquid was possible but a significant decrease in the conversion after the fourth cycle was observed. This decrease was attributed to leaching of the Ru complex, which was also confirmed by atomic absorption analysis. To prevent leaching and thus improve recyclability, Lin and co-workers prepared two polar phosphonic acid-derived Ru-BINAP systems 26 and 27 for asymmetric hydrogenation of β-keto esters in ionic liquids (Scheme 1.6).
Several β-keto esters were hydrogenated with high enantioselectivities (up to 99.3% ee) using both catalysts 26 and 27 in [bmim]BF4 and [bmim]PF6 as well as in [bdmim]NTf2. The catalysts performed best in a mixture of ionic liquid and methanol. Products were simply extracted with degassed hexane and the ionic liquid was reused in the next experiment, after drying. Direct current plasma spectroscopy showed that only very little ruthenium leeched into the organic phase (between 0.01 and 0.04%, depending on ionic liquid used). It was possible to recycle the catalyst in ionic liquid four times with only small or no decrease in enantioselectivity and conversion. Enantioselectivities with polar catalyst were comparable with unmodified Ru-BINAP system in ionic liquids.
Lin and co-workers later studied the effect of substitution of Ru-BINAP catalytic system in more detail. They found that polar substituents led to a decrease in enantioselectivity in methanol, but the P(O)(OH)2 group was more enantioselective in a mixture of [bmim]BF4/MeOH.
Modified Ru-BINAP catalyst 28 and 29 for hydrogenation of β-keto esters was also developed by Lemaire and co-workers (Figure 1.6). These catalysts, however, were slightly less active. Hydrogenation of ethyl acetoacetate proceeded with enantioselectivity (90% ee) and only one recycling was performed.
Chan and co-workers showed that ionic tagging is not absolutely necessary for highly enantioselective and recyclable ruthenium catalysts. They showed that even the polar bipyridine derivative 30 can be e?ciently recycled four times without loss of activity (Figure 1.6). In the mixture [bmim]PF6/MeOH, methyl acetoacetonate was reduced with 99% conversion and 98% ee and in the ?fth cycle conversion was still 96% and enantioselectivity 93%.
1.3.2 Catalysts for Enantioselective C–C and C–Heteroatom Bond Formation
A chiral vanadyl salen complex was tagged with the imidazolium ion. Complex 31 catalyzed the asymmetric cyanosilylation of benzaldehyde in [bmim]PF6. The enantioselectivity of the reaction (57% ee) was signi?cantly lower than that with unmodified vanadyl ligand (89% ee). However, the catalytic activity of complex 31 was considerably higher than that of the unmodified catalyst as well as the catalyst anchored on silica, activated carbon and single wall carbon nanotube (Scheme 1.7).
Moreau and co-workers prepared imidazolium-tagged camphorsulfonamide ligands for titanium-promoted asymmetric Et2Zn addition to benzaldehyde (Scheme 1.8). Alkylation of benzaldehyde in the presence of the titanium complex of 32 was performed in CH2Cl2. Conversion were over 99% in all cases, but enantioselectivities were only moderate (65% ee). Separation of the product from the catalyst was achieved by evaporation and hydrolysis followed by extraction with diethyl ether, in which the catalyst was not soluble. The catalytic system was used four times without any change in catalytic activity and enantioselectivity.
Moreau and co-workers continued the development of titanium-mediated addition of diethylzinc to aldehydes by preparation of ion-tagged BINOL ligand 33 (Scheme 1.8). The ligand 33 afforded Et2Zn addition with 82% ee. The same result was obtained also with unmodified BINOL. The reaction was performed in CH2Cl2 but the catalyst was recyclable three times with same activity and enantioselectivity.
The fact that dialkylzinc additions to aldehydes can also be catalyzed by simple amino alcohols motivated Lombardo and co-workers to design an amino alcohol catalyst with an ionic tag. They selected diphenylprolinol for its availability and ease of modification. An ionic tag was introduced by alkylation of pyrrolidine nitrogen. Envisaged problems with carbene formation in imidazolium cations prompted them to use the simple trialkylammonium cation instead. These considerations led to three amino alcohols 34 with different spacer length. Choice of ionic liquid was also motivated by higher stability towards basic diethylzinc. Thus addition of diethylzinc to benzaldehyde proceeded best in [bmpy]NTf2 giving product in 80% yield and with 89% ee. The different length of alkyl spacer did not affect chemical yields but it had a profound effect on enantioselectivity. Catalysts 34a (n = 3) and 34c (n = 6) were less selective (72 and 80% ee, respectively). The striking effect of the ionic tag was demonstrated by comparison with N-methyldiphenylprolinol, with which the reaction proceeded with good conversion but only with 38% ee. Ethylation of benzaldehyde was run 10 times without any decrease in chemical yield or enantioselectivity (Scheme 1.9). The authors also developed an efficient recycling procedure. Zinc salts were removed from the ionic liquid by aqueous EDTA solution. The product was extracted with diethyl ether and the ionic liquid with catalyst was reused after drying. A range of aldehydes were ethylated by this method, giving products typically in over 90% yield and 90% ee.
(Continues...)
Excerpted from Enantioselective Homogeneous Supported Catalysis by Radovan ?ebesta. Copyright © 2012 Royal Society of Chemistry. Excerpted by permission of The Royal Society of Chemistry.
All rights reserved. No part of this excerpt may be reproduced or reprinted without permission in writing from the publisher.
Excerpts are provided by Dial-A-Book Inc. solely for the personal use of visitors to this web site.