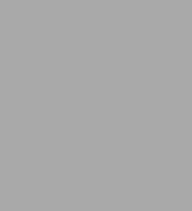
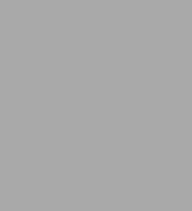
Hardcover(Edition. ed.)
-
PICK UP IN STORECheck Availability at Nearby Stores
Available within 2 business hours
Related collections and offers
Overview
About the Author
Read an Excerpt
Energy Crops
By Nigel G Halford, Angela Karp
The Royal Society of Chemistry
Copyright © 2011 Royal Society of ChemistryAll rights reserved.
ISBN: 978-1-84973-032-7
CHAPTER 1
Energy Crops: Introduction
ANGELA KARP AND NIGEL G. HALFORD
Departments of Plant and Invertebrate Ecology and Plant Science, Centre for Bioenergy and Climate Change, Rothamsted Research, Harpenden, Herts AL5 2JQ, UK
1.1 Introduction
Two major events that impacted significantly on the development of humankind involved the use of plants: our ability to make fire and our change from being hunter-gatherers to food-producers. The exact dates in which these advances first occurred are subject to debate but it is certain that they occurred in this order. Estimates suggest that agriculture arose some 10 000 years ago, whilst the control of fire may date back some 790 000 years. As food production became more efficient, it became possible for larger numbers of people to live together. Human populations expanded and civilisations were born. During this expansion, the requirement for plants to provide fuel was not in conflict with food production. Rather, this requirement diminished as alternative sources of energy were developed. As a result, twenty-five years ago, although plants were still being used for fuel in underdeveloped regions of the world, it was oil, coal, natural gas and nuclear power that together fulfilled most of the world's energy needs.
Within a remarkably short timeframe, a major change then took place. The first steps of this process can be traced to the first oil crisis of the 1970s, when a sudden rise in the price of oil led to the first push for the development of renewable energies. In addition to food production, many governments supported the development of novel nonfood crops (see Chapters 11–18). These crops were harvested and combusted to produce heat and power, a contribution that continues today. However, a drop in oil prices in the 1990s stemmed enthusiasm and, other than the production of bioethanol in Brazil (see Chapter 5), bioenergy markets remained small.
A culmination of events then precipitated a "green energy" boom. Oil prices spiked and there was increasing concern over energy security. Moreover, numerous reports, particularly from the Intergovernmental Panel on Climate Change (IPCC) and from Nicholas Stern, focused attention on the substantial cost to humankind of not acting to reduce the current rate of increase in greenhouse gas (GHG) emissions. As the use of fossil fuels is a major contributor to climate change, the need for alternative sources of energy, which save carbon and are renewable, was placed quickly and firmly back at the top of global agendas.
There are many possible alternatives to fossil fuels, particularly for heat and power generation, e.g. wind, hydro, solar, as well as plant biomass, all of which are expected to play a role. However, there are few alternatives to replace transport fuels (e.g. electric, hydrogen). As the number of vehicles on the roads is continually rising, it is clear that, unless emissions from the transport sector can be curbed, they will counter any reductions achieved by other sectors. Combined with the desire of some nations to reduce their reliance on the fuel supplies of a few major producers, this resulted in a swift, strong push to increase the production of liquid transport fuels from crops and many food crops were exploited for this purpose (see Chapter 2–10). Simultaneously, the need for fossil-fuel substitutes for a whole range of products that currently rely on the refining of oil became apparent to the chemical industries.
As a consequence of these environmental and political drivers, three new markets have emerged for plants, all of which are potentially huge: bioenergy, biofuels and biorenewable materials (Figure 1.1). However, securing sufficient food for future populations remains a major challenge, particularly in the face of climate change. To balance all of these demands on plants will require little short of another revolution. One decade into this millennium, major challenges face humankind. "A Perfect Storm" were the words adopted by Professor Beddington, the UK government's chief scientific adviser, to draw attention to this recently. He pointed specifically to research that indicated that by 2030 "a whole series of events come together": The world's population will rise from 6bn to 8bn (33%); demand for food will increase by 50%; demand for water will increase by 30% and; demand for energy will increase by 50%.
Whilst crops certainly hold solutions to these challenges, they are also part of the problem. Agriculture is a major user of resource, including energy from fossil fuels, and is also another major contributor to GHG emissions. Research has drawn attention to the fact that producing energy from crops could have the opposite effect to what is intended, if the energy inputs exceed the carbon benefits and if the GHG emissions associated with any new cultivation for displaced food production are not taken into account.
In this book, the potential contributions of different energy crops will be reviewed within the context of the different and sometimes conflicting challenges and drivers that are currently acting. By way of introduction, this chapter will introduce various bioenergy terms, outline how feedstock from energy crops can be converted into energy, briefly define the different types of energy crops and finally draw attention to the main components of the debate surrounding energy crop production. The principle aim will be to introduce the topics and not to comprehensively review them, as the subsequent chapters will provide the details.
1.2 Biorenewables, Bioenergy and Biofuel Definitions
"Biorenewables" is an all-embracing term that covers the production of heat, power, transport fuel and other products from organic matter of recent (as distinct from fossil) origin. These converge in the "biorefinery" concept, which can be defined as the sustainable coproduction of a spectrum of biobased products (food, feed, materials, chemicals) and energy (fuels, power, heat) from biomass. However, not all biorenewable products are derived from biorefining per se. The need for more sustainable products with reduced carbon footprints has led to the development of many new supply chains from nonfood crops and wastes.
In this book we will confine our discussions to the production of renewable energy from biological materials, or "bioenergy". In practice, this term is sometimes used with reference only to renewable heat and power and sometimes also to include renewable transport fuels. Here, we adopt an earlier definition and take it to mean the production of any renewable energy, reserving the use of the term "biofuels" for renewable liquid transport fuels only. Biofuels can be subdivided into "bioethanol" made from grain and sugar crops, which provides a substitute for petrol, and "biodiesel", made from vegetable oil, which is a substitute for diesel (see Sections 1.3.3 and 1.3.4).
Energy crops can be grouped on the basis of the main market their production is targeted at (e.g. "biofuel crops") and on the type of feedstock that they provide for conversion (e.g. "biomass crops"). This is then further qualified by taking into account the different conversion processes that can be applied and by virtue of the fact that more than one type of energy source can be obtained from the same crop. Thus, the term "biomass crops" is most often used with reference to growing crops for the purpose of producing total biological mass as a feedstock, whilst "lignocellulosic crops" refers more specifically to use of the cell wall components of plants. "Bioethanol crops" and "biodiesel crops" refer to crops grown for production of bioethanol and biodiesel, respectively, and so on. There are also secondary classifications of "first" and "second" generation, depending upon whether conversion is based on simple sugars or more complex lignocelluloses as the energy source (see below). Whilst this book is not concerned with detailed descriptions of the conversion processes (a topic to be covered by another volume in this series), it is important to outline what they are in order to have clarity over the specific energy-crop system being referred to at any one time.
1.3 Converting Crops to Energy
There are four main routes to energy conversion that can be summarised as: direct combustion, thermal conversion (pyrolysis, gasification), biological conversion (anaerobic digestion, fermentation) and chemical conversion (transesterification).
1.3.1 Direct Combustion
Direct combustion is the oldest and still the most commonly used route for converting biomass to heat, power and combined heat and power (CHP). It is applicable at all scales from domestic to industrial, using boilers that range from small domestic stoves (1 to 10 kW) to the largest devices used in power and CHP plants (>5 MW). Biomass of many different forms can be used, including wood chip, pellets and different straws. The most efficient combustion is achieved when dedicated biomass boilers are used. However, solid biomass particles can also be mixed with coal in cofiring or cogeneration, enabling the large power-generation industries to reduce their carbon footprint by incorporating biomass as a percentage of their feedstock.
1.3.2 Thermal Conversion
In thermal conversion processes, high temperatures are used but energy is not produced directly from biomass. Instead, the biomass is converted to energy carriers such as synthetic gases, oil or methanol that have higher energy densities (and thus lower transport costs) and/or more predictable and improved combustion characteristics. The two main thermochemical processes, pyrolysis and gasification, differ with respect to the presence/absence of oxygen.
Pyrolysis occurs in the complete absence of oxygen at temperatures in the range of 400–800 °C. During this process, most of the cellulose and hemi-cellulose and part of the lignin, which together make up the lignocellulosic fraction, disintegrate and form gases. As these gases cool, some of the vapours condense to form bio-oil, which has potential as a substitute for fuel oil and as a feedstock for synthetic gasoline or diesel production. The remaining biomass, mostly comprised of lignin, forms charcoal.
Gasification requires partial oxidation and temperatures of around 800 1C. The biomass is partially burned to form "producer gas" and charcoal. The carbon dioxide (CO2) and water (H2O) in the producer gas are chemically reduced by the charcoal to form carbon monoxide (CO) and hydrogen (H2). Producer gas contains 18–20% H2, 18–20% CO, 8–10% CO2, 2–3% methane (CH4), trace amounts of higher hydrocarbons (e.g. methane and ethane), water, nitrogen (if air is used as the oxidising agent) and various contaminants such as small char particles, ash, tars and oils. The partial oxidation in the gasification process can be carried out using air, oxygen, steam or a mixture of these. When air is used, a low heating value gas is produced that is suitable for use in boilers, engines and turbines but not for transporting in pipelines, because of the low energy density. Gasification with oxygen or steam produces a medium heating value gas, which is suitable for limited pipeline distribution, as well as a synthesis gas or "syngas" (typically 40% CO, 40% H2, 3% CH4 and 17% CO2, dry basis). Syngas can be used to make methanol, ammonia and diesel using Fischer–Tropsch synthesis,10 first developed by F. Fischer and H. Tropsch in 1923.
1.3.3 Biological Conversion
There are two main forms of biological conversion: anaerobic digestion and fermentation. Both are well-developed technologies.
Anaerobic digestion (AD) is the breakdown of organic materials by bacteria in the absence of oxygen. Almost any organic material can be processed, including waste paper, grass cuttings, food waste, industrial effluents, sewage and animal waste. The result is a biogas made up of around 60% methane and 40% CO2. Biogas can be burnt to generate heat or (once it is scrubbed) electricity. It can also be used as a biofuel. A solid and liquid residue called digestate is also produced, which can be used as a soil conditioner. The amount of biogas and the quality of digestates obtained vary according to the feedstock used. More biogas will be produced if the feedstock is putrescible, which means it is more liable to decompose. The use of sewage and manure produces less biogas as the animal that produced it has already removed some of the energy content.
Fermentation, followed by distillation, is the biological conversion process used for converting sugars to ethanol or, depending on the microbial strain, other low molecular weight alcohols. Most ethanol fermentation is based on Baker's yeast (Saccharomyces cerevisiae), which requires simple (monomeric) sugars as raw material. Conventional yeast fermentation produces 0.51 kg of ethanol from 1 kg of any of the C6 sugars, such as glucose and mannose, or sucrose. However, not all feedstocks contain simple sugars. Starch, for example, is a polymer and when starch is used a hydrolysis step is first required to break it down into simple sugars for fermentation. Bioethanol production from starch is now well established in the USA, with maize (Chapter 3) being the major raw material.
Polymeric carbohydrates are also present in all cell walls of the plant and potentially provide the most abundant source of carbon for bioenergy and biofuel production available on earth. However, in this form the sugars are not readily accessible, existing in the form of fibres, sometimes interlinked with lignin, and additional steps of pretreatment and hydrolysis are required to release the sugars for fermentation. The cell wall polymers of wheat are described in Chapter 2.
Fermentation and distillation to produce ethanol from sugar have been at the heart of the brewing and of the wine and spirit industries for a long time in human history. The same well-developed and efficient procedures have been used effectively to produce alcohols that can be used as fuels for internal combustion engines. This is well established in Brazil using sugar from sugarcane (Chapter 5), and is being developed in the USA using sweet sorghum (Chapter 4). When used as a biofuel, ethanol is blended with gasoline. The percentage blend depends on the engine type: a 10% blend (E10) can be used by most engines, whereas an 85% blend (E85) requires specialised flexifuel vehicles.
1.3.4 Chemical Conversion
The main chemical conversion process is transesterification which results in the production of biodiesel; effectively, biodiesel is the fatty acid methyl esters (FAME) produced from different oil-containing crops. In fact, lipids (e.g. from algae (Chapter 18)) and oils, either directly produced by crops or derived from processed vegetable oil from the food industry, can all be used as feedstock for conversion into biodiesel.
(Continues...)
Excerpted from Energy Crops by Nigel G Halford, Angela Karp. Copyright © 2011 Royal Society of Chemistry. Excerpted by permission of The Royal Society of Chemistry.
All rights reserved. No part of this excerpt may be reproduced or reprinted without permission in writing from the publisher.
Excerpts are provided by Dial-A-Book Inc. solely for the personal use of visitors to this web site.