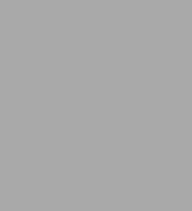
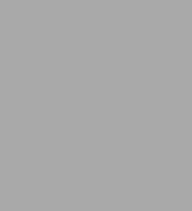
Paperback(New Edition)
-
SHIP THIS ITEMIn stock. Ships in 1-2 days.PICK UP IN STORE
Your local store may have stock of this item.
Available within 2 business hours
Related collections and offers
Overview
Product Details
ISBN-13: | 9780521536332 |
---|---|
Publisher: | Cambridge University Press |
Publication date: | 08/25/2005 |
Edition description: | New Edition |
Pages: | 386 |
Product dimensions: | 7.44(w) x 9.69(h) x 0.79(d) |
About the Author
Read an Excerpt
Cambridge University Press
0521829364 - Environmental Change - Key Issues and Alternative Perspectives - by Frank Oldfield
Excerpt
Chapter 1
Defining and exploring the key questions
1.1 Global changes present and past
Any of our ancestors living a full three score years and ten in Western Europe some 11 600 years ago would have experienced, during their life time, truly remarkable changes in climate. Evidence from that time shows that the main changes took place over a period of 50 years at most. Although different lines of evidence give different figures for the degree of warming, it would be difficult to argue for an increase of less than 4 °C in mean annual temperature over much of Western Europe. In many areas, the shift would have been substantially greater. Parallel changes varying in nature and amplitude, but often synchronous in timing, took place over much of the Earth. The stratigraphic signal of these changes in the records from sediments and ice cores marks the transition from glacial times to the opening of the Holocene, the interglacial in which we live. Here then, was a period of rapid 'global change'. We may infer from this that there is nothing so very special about what we now think of as global change, that is, the current and impending changes in the Earth system driven by human activities. We would be quite wrong. The changes under way at the present day are of a different kind. At this stage, we need consider only three key differences:
- The rate of change in atmospheric CO2 concentrations exceeds the mean rate during glacial-interglacial transitions by one to two orders of magnitude (Raynaud et al., 2003).
- The human population is now many orders of magnitude greater than it was at the opening of the Holocene.
- The degree to which the full range of human activities has transformed the world and the way it functions, especially over the last 50 years, has created a biosphere with no past analogues. Humans have become agents of change with diverse and increasing impacts on almost every aspect of the Earth system.
Another way of highlighting the unique nature of the global changes currently under way is to consider present-day and projected greenhouse-gas concentrations in relation to the values typical of the Earth system over the last four glacial cycles. Figure 1.1 shows how far outside this envelope of recent natural
Image not available in HTML version |
Figure 1.1 Past, present and the range of projected future atmospheric concentrations of carbon dioxide and methane. (Modified from Alverson et al., 2001.)
variability current and projected future atmospheric concentrations of carbon dioxide and methane lie. Trying to tease out the climatic and broader environmental implications, as these values surge beyond what were their natural limits over the last four glacial cycles, will be a recurrent theme throughout the book. Contemporary and future global changes serve as substantive themes in their own right. In addition, by sharpening the focus on those lines of inquiry most likely to shed light on the processes involved in present and future changes, they also provide criteria of relevance in relation to evidence from the past.
1.2 Earth-system science
Central to any exploration of environmental change is the concept of an integrated Earth system of which climate is a part and in which human activities now play a major, integral role (Figure 1.2). As we shall see from numerous examples throughout the book, climate interacts with all the other components of the Earth system in complex ways involving multiple feedbacks between ocean, terrestrial biosphere, cryosphere and atmosphere. To view climate as a self-contained atmospheric component of global change, separated from all the other processes that interact with it, is unrealistic. Equally, to view human activities as separated from, or running counter to the 'natural' world, rather than as key agents in the processes that are transforming the Earth system, would be to revive an earlier and now totally inappropriate conceptual framework.
It follows from the above that any realistic scientific appraisal of present and future global change, indeed the whole field of study that we are addressing under the heading 'global change' rests on the emerging field of Earth system science. Using the definition given in Steffen et al. (2004): 'The Earth System has come to mean, broadly, the suite of interacting physical, chemical, and biological global-scale cycles (often called biogeochemical cycles) and energy fluxes which provide the conditions necessary for life on the planet.'
A comprehensive account of Earth-system science lies outside the scope of this book. Nevertheless, it forms the framework within which the key questions, research themes and case studies will be considered. Some of the main processes involved and some generic characteristics of the Earth system and its major, interacting components, including human populations, are set out below.
1.2.1 Key Earth-system processes
Central to any understanding of how the Earth system operates and how it changes on timescales of greatest relevance to future human welfare, are the processes that control changes in the fluxes of energy within the atmosphere and between atmosphere, hydrosphere, cryosphere and biosphere. These in turn largely control climate. Figure 1.3 is a schematic representation of the main processes driving change in the climate system. Even at this level of generalisation, many processes and transformations are involved. Several questions arise from this schematic diagram. What modulates changes through time in the external input of energy through solar radiation? What are the main ways in which the various processes shown in Figure 1.3 can be changed? How do the most important mechanisms redistributing incoming energy around the globe vary through time?
Solar energy received at the top of the atmosphere averages approximately 1365 W m-2. Only since 1980 has it been possible to make direct measurements of the way in which this solar 'constant' actually varies through time as a result of quite a diverse range of processes (see e.g. Beer et al., 2000). For periods further back in time and on longer timescales, changes in incoming solar radiation have to be inferred from proxy measurements (see Section 4.3.2) and from calculations based on the behaviour of the Solar System (see 4.3.1). Figure 4.6 shows aspects of
Image not available in HTML version |
Figure 1.2 A wiring diagram of the Earth system. Key boundary conditions and major external forcings are at the top and left-hand side of the diagram. Human activities are indicated down the right-hand side. Some of the main environmental archives recording past changes are shown along the bottom. (Based on Schellnhuber, 1999.)
Image not available in HTML version |
Figure 1.3 Schematic diagram of the main components of the climate system, showing many of the linkages and exchanges that play an important role in climate variability. (Based on Bradley, 1999.)
the reconstructed variability on a range of timescales from sub-annual to multi-millennial. As the timescale lengthens, the importance of low-frequency changes in the Earth's orbit increases. These changes are now considered the likely 'pace-makers' of the major switches between glacial and interglacial conditions that dominate the recent climate history of the Earth (see 3.4.1 and 5.2.1).
Although the existence of significant links between solar variability and climate change on Earth is generally accepted, the extent to which it dominates other factors is controversial. Moreover, the variations in energy received at the top of the atmosphere are so small in relative terms that much uncertainty surrounds the mechanisms responsible for the links on all timescales.
The above account deals only with the incoming arrow at the top of Figure 1.3. We must next consider briefly the major forces responsible for redistributing the energy received around the globe - the large-scale systems of atmospheric and ocean circulation. These are shown schematically in Figures 1.4 and 1.5. They serve here mainly as points of reference for subsequent sections of the text, though several initial observations can be made. The response times of the two types of system vary across many orders of magnitude. The radiative balance of the atmosphere equilibrates in a matter of hours or days, whereas in the deep ocean it may take hundreds of years. The ocean provides both storage and transport of heat on a massive scale. Coupling through time between these two systems is complex. Both systems are strongly influenced by the actual configuration of land and sea, leading to major contrasts in circulation between, for example, the atmosphere in the northern and southern hemispheres and between the Atlantic and Pacific oceans. In the case of the atmosphere, one of the main contrasts arises from the different thermal capacity of the land masses and oceans, leading to the dominance of monsoon-type climate systems in lower latitudes in many parts of the northern hemisphere. In the case of the oceans, the main contrasts arise from the dominance of the Atlantic by the system of meridional overturning circulation (see, e.g., Ganachaud and Wunsch, 2000.) and from the uniquely powerful westerly circum-polar flow in the southern
Image not available in HTML version |
Figure 1.4 A schematic diagram of the main components of atmospheric circulation. H - high pressure; L - low pressure; ICTZ - inter-tropical convergence zone.
hemisphere. The major systems of persistent energy flux (in the atmosphere: the westerlies, the tropical anticyclone cells, the equatorial Hadley cell; in the oceans: the thermohaline circulation for example) all include complex subsystems that vary continuously in terms of their spatial domains and relative strength. All aspects of each system are subject to modulation, not only by the strength and distribution of the external energy flux from the Sun, but also by feedback processes linking the atmosphere and oceans and operating between them and the other components of the Earth system.
The last of the above points leads to a preliminary review of some of the additional feedback processes involved in regulating the fluxes of energy in the Earth system and the all-pervasive climate component. At the interface between land and atmosphere, one of the most important factors is surface albedo, which controls the amount of incoming radiation that is retained or reflected back into the atmosphere. The reflected fraction can vary from around 80% where snow cover is continuous, to around 40% for un-vegetated hot deserts and to well below 20% in densely forested regions. Changes in climate that affect snow and ice cover and vegetation thus lead to changes in albedo that may reinforce or counter the initial change. Where ice extent varies through time over the ocean, there are at least equally significant consequences. Not only does this change the
Image not available in HTML version |
Figure 1.5 The main features of ocean circulation. NADW - North Atlantic deep water; AABW - Antarctic bottom water. The circulation within the Atlantic is marked by southerly flowing deep water and northerly flowing upper-level water. Together these comprise the meridional overturning circulation system, which, in turn exerts a dominant influence on the thermohaline circulation that links the Atlantic circulation to that in the Indian and Pacific oceans (Based on WMO/ WCRP sources.)
albedo and thermal capacity of the surface, it also modifies salinity, hence the density gradients within the water column. This can affect heat exchange between surface and deep water and the pattern of ocean circulation. These, in turn, have important implications for climate.
Feedbacks arise from processes within the atmosphere and the oceans as well as in the boundary layer between the two. Clouds have the effect of both screening the Earth from incoming radiation and reflecting back to Earth outgoing long-wave radiation. The balance between the two varies for any given cloud type and the altitude at which it develops. Indeed, the role of clouds in the climate system continues to be notoriously difficult both to measure and to model. Dust and aerosols also serve to absorb and scatter incoming radiation. Over the last two decades especially, the role of greenhouse gases and more recently aerosols has attracted great attention. Water vapour, carbon dioxide and methane are the most important and commonly considered greenhouse gases. Recent, rapid increases in the atmospheric concentration of the latter two as a result of human activities are beyond doubt. The rate of any future increases remains uncertain. The precise implications of any given increase in terms of surface warming remain controversial, partly because quantifying the past and likely future role of water vapour as a greenhouse gas has proved so elusive. The whole question of greenhouse feedback will thread through later chapters.
Simply to calculate the implications of increased CO2 emissions for atmospheric concentrations, without any regard for wider consequences, requires an understanding of the carbon cycle. This involves every major component of the Earth system. There are major carbon reservoirs in the terrestrial biosphere and soils as well as in the oceans and atmosphere (see Figure 5.3). Fluxes between these reservoirs are extremely difficult to calculate on a global scale even for the present day, let alone the past or future. In consequence, current best estimates of the main components of the present-day global carbon budget are subject to large uncertainties. The main controls on the system include complex biological and geological processes that interact on timescales ranging from seasonal through inter-annual and decadal to multi-millennial.
Despite the complexity of the Earth system, there are some generic characteristics of environmental systems that can be introduced at the outset and these are considered next.
1.2.2 Some generic characteristics of environmental systems
Although a great deal of research in environmental science still uses concepts of simple cause and effect, as well as linear approximations to forcing-response relationships, we are increasingly obliged to realise that the Earth system does not behave in this way. Most of the changes that we seek to understand involve non-linear responses to whatever set of processes influences them; that is to say, there is no simple proportionality between the driving force and the response. Nor is there necessarily any immediately detectable response to forcing. What we often see is a delayed response reflecting the transgression of some kind of threshold in the system. Below the threshold, the response may be within the range of variability typical of the unforced state. Once the threshold is crossed, the response may then be out of all proportion to the forcing. This is due to the power of internal feedback mechanisms that may interact to amplify the effects of the original forcing, once the threshold beyond which they can maintain the unforced state is crossed. Thresholds thus combine with the intrinsic (i.e. internal), non-linear system dynamics to create lags, hysteresis (whereby the trajectories that systems follow as they are perturbed from an initial state differ from those followed as they recover from the perturbation), and disproportionate responses. This is a dangerous combination, for it confronts us with the possibility that there may be critical thresholds in the future of which we have, as yet, no warning, but which, once crossed, may herald major, non-linear responses. Part of the challenge is therefore to identify crucial thresholds and characterise key feedback mechanisms in the Earth system so that those posing the greatest dangers to human populations may be avoided.
This task is made all the more difficult by other features of the Earth system characterised as emergent behaviour and contingency. Many components of the Earth system at all spatial scales, when unperturbed, tend to evolve towards increasing internal order, complexity and differentiation. For example, within a developing ecosystem, the minor environmental variations that may exist on a newly exposed land surface evolve divergently to become expressed as spatial variations in soil profiles, vegetation and food webs. One implication of emergent behaviour is that many features of the Earth system that we see at the present day are contingent upon their histories. This adds greatly to the difficulties involved in developing quantitative predictive statements from a limited number of studies.
1.3 The key issues: a preliminary analysis
What are the first-order issues of greatest importance to environmental scientists addressing the challenge of global change? The following paragraphs attempt to define and briefly explore these in light of the foregoing introduction to Earth system science and in terms of the major research issues that each one raises. They introduce the main themes addressed in the remainder of the book.
1.3.1 Global climate change
What will be the amplitude and rate of global climate change over the next century and beyond?
To address this question, we need to consider further the biophysical forcing and feedback mechanisms that control the energy budget of the Earth system. These include natural, external forcing mechanisms such as solar variability and volcanic activity, as well as forcing and feedback processes strongly impacted by human activities: for example, atmospheric greenhouse gases, dusts and aerosols. The need to characterise and quantify human impacts on these latter, and also on other chemical species that strongly influence the Earth system, leads to a consideration of industrialisation and land cover changes since both are key drivers of changes in atmospheric composition. The way in which this complex combination of natural and anthropogenic influences affects climate at any point on the Earth's surface is partly a function of the way in which the atmosphere and the oceans redistribute energy around the globe. The processes involved in this redistribution are not only strongly interactive, they are also influenced by feedback mechanisms that link them to the terrestrial biosphere. Thus the whole Earth system is intimately involved. Moreover, all the human activities that come into play reflect cultural, economic, technological and political processes operating on a range of scales from personal to global. For example, future rates of increase in atmospheric greenhouse gases will depend not only on the biophysical processes by which CO2, methane and the other gases are processed and ultimately transformed or sequestered in components of the Earth system; they will reflect economic and technologically based decisions about, for example, energy production and the reduction of emissions. Human priorities and aspirations are just as crucial as trace-gas atmospheric residence times or the rate at which sediments in the deep ocean can provide long-term carbon storage (see 13.1.2).
We can study the way most of these processes operate and interact in the present day, though many methodological difficulties remain and major uncertainties persist when we attempt a fully quantitative analysis, or a level of explanation that captures all the interactions. We can also reconstruct the operation of many of the key processes and interactions for the past. What is much more difficult is to predict with confidence, or even with statistically quantifiable uncertainty, what will happen in the future. Even developing conditional projections or attaching degrees of probability to alternative future scenarios has proved to be extremely challenging. Poorly understood thresholds and inadequately quantified non-linearities in the biophysical realm, coupled with unpredictable discontinuities in human affairs, make all attempts to look into the future to a greater or lesser degree speculative. Yet, paradoxically, it is the imperative nature of that forward look that now drives much of the science upon which this book draws. Increasingly, we have come to rely on future projections, scenarios or 'story lines', each based on a plausible set of trajectories for the main processes responsible for future climate change.
1.3.2 Sea-level change
What will be the likely response of sea-level to global climate change?
Our understanding of the climate system and its future behaviour is vital to any estimate of the way in which sea-level may change in the future. A recent, at least century-long rise in global mean sea-level is well documented and can be ascribed in part to the warming, hence expansion, of the surface layers of the ocean (the so-called steric effect) and partly to the melting of glaciers. In order to assess the likely future rate and amplitude of any further sea-level rise, we need to be able to quantify better the recent trends and ascribe them more precisely to the main processes involved. Complementary research on the influence of climate change on sea-level in the past is also necessary. The links between climate and sea-level are complex and even the simplest cause-effect relationships are subject to time-lags, hence the value of a longer time perspective. Beyond this, that essential look into the future will, as in the case of climate change, remain a matter of alternative scenarios, each dependent on a trajectory of climate change and a series of calculations designed to estimate the effects of the climate change on the main controls of sea-level - ocean surface temperature and the mass balance of both polar and temperate ice.
When we move on one step further and attempt to estimate the likely effect of a given rise in global sea-level on a specific site or region, we need to know how to translate a mean rise world-wide into impacts on a particular coastline, with a specific morphology, tidal regime and sediment budget. We also need to be able
© Cambridge University Press