Introductory Chemical Engineering Thermodynamics, Second Edition, helps readers master the fundamentals of applied thermodynamics as practiced today: with extensive development of molecular perspectives that enables adaptation to fields including biological systems, environmental applications, and nanotechnology. This text is distinctive in making molecular perspectives accessible at the introductory level and connecting properties with practical implications.
Features of the second edition include
- Hierarchical instruction with increasing levels of detail: Content requiring deeper levels of theory is clearly delineated in separate sections and chapters
- Early introduction to the overall perspective of composite systems like distillation columns, reactive processes, and biological systems
- Learning objectives, problem-solving strategies for energy balances and phase equilibria, chapter summaries, and “important equations” for every chapter
- Extensive practical examples, especially coverage of non-ideal mixtures, which include water contamination via hydrocarbons, polymer blending/recycling, oxygenated fuels, hydrogen bonding, osmotic pressure, electrolyte solutions, zwitterions and biological molecules, and other contemporary issues
- Supporting software in formats for both MATLAB® and spreadsheets
- Online supplemental sections and resources including instructor slides, ConcepTests, coursecast videos, and other useful resources
Introductory Chemical Engineering Thermodynamics, Second Edition, helps readers master the fundamentals of applied thermodynamics as practiced today: with extensive development of molecular perspectives that enables adaptation to fields including biological systems, environmental applications, and nanotechnology. This text is distinctive in making molecular perspectives accessible at the introductory level and connecting properties with practical implications.
Features of the second edition include
- Hierarchical instruction with increasing levels of detail: Content requiring deeper levels of theory is clearly delineated in separate sections and chapters
- Early introduction to the overall perspective of composite systems like distillation columns, reactive processes, and biological systems
- Learning objectives, problem-solving strategies for energy balances and phase equilibria, chapter summaries, and “important equations” for every chapter
- Extensive practical examples, especially coverage of non-ideal mixtures, which include water contamination via hydrocarbons, polymer blending/recycling, oxygenated fuels, hydrogen bonding, osmotic pressure, electrolyte solutions, zwitterions and biological molecules, and other contemporary issues
- Supporting software in formats for both MATLAB® and spreadsheets
- Online supplemental sections and resources including instructor slides, ConcepTests, coursecast videos, and other useful resources
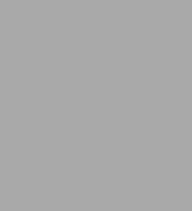
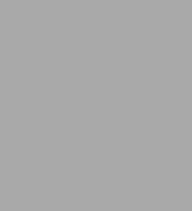
eBook
Available on Compatible NOOK devices, the free NOOK App and in My Digital Library.
Related collections and offers
Overview
Introductory Chemical Engineering Thermodynamics, Second Edition, helps readers master the fundamentals of applied thermodynamics as practiced today: with extensive development of molecular perspectives that enables adaptation to fields including biological systems, environmental applications, and nanotechnology. This text is distinctive in making molecular perspectives accessible at the introductory level and connecting properties with practical implications.
Features of the second edition include
- Hierarchical instruction with increasing levels of detail: Content requiring deeper levels of theory is clearly delineated in separate sections and chapters
- Early introduction to the overall perspective of composite systems like distillation columns, reactive processes, and biological systems
- Learning objectives, problem-solving strategies for energy balances and phase equilibria, chapter summaries, and “important equations” for every chapter
- Extensive practical examples, especially coverage of non-ideal mixtures, which include water contamination via hydrocarbons, polymer blending/recycling, oxygenated fuels, hydrogen bonding, osmotic pressure, electrolyte solutions, zwitterions and biological molecules, and other contemporary issues
- Supporting software in formats for both MATLAB® and spreadsheets
- Online supplemental sections and resources including instructor slides, ConcepTests, coursecast videos, and other useful resources
Product Details
ISBN-13: | 9780132901093 |
---|---|
Publisher: | Pearson Education |
Publication date: | 02/06/2012 |
Series: | Prentice Hall International Series in the Physical and Chemical Engineering Sciences |
Sold by: | Barnes & Noble |
Format: | eBook |
Pages: | 912 |
File size: | 75 MB |
Note: | This product may take a few minutes to download. |
Age Range: | 18 Years |
About the Author
Carl T. Lira is Associate Professor in the Department of Chemical Engineering and Materials Science at Michigan State University. He teaches thermodynamics at all levels, chemical kinetics, and material and energy balances. He has been recognized with the Amoco Excellence in Teaching Award and multiple presentations of the MSU Withrow Teaching Excellence Award. He holds a Ph.D. from the University of Illinois.
Read an Excerpt
Preface
"No happy phrase of ours is ever quite original with us; there is nothing of our own in it except some slight change born of our temperament, character, environment, teachings and associations."Thank you for your interest in our book. We have developed this book to address ongoing evolutions in applied thermodynamics and computer technology. Molecular perspective is becoming more important in the refinement of thermodynamic models for fluid properties and phase behavior. Molecular simulation is increasingly used for exploring and improving fluid models. While many of these techniques are still outside the scope of this text, these new technologies will be important to practicing engineers in the near future, and an introduction to the molecular perspective is important for this reason. We expect our text to continue to evolve with the chemical engineering field.
Mark Twain
Computer technology has made process simulators commonplace in most undergraduate curriculums and professional work environments. This increase in computational flexibility has moved many of the process calculations from mainframe computers and thermodynamic property experts to the desktop and practicing engineers and students. This increase in computational ability also increases the responsibility of the individuals developing process simulations to choose meaningful models for the components in the system because most simulators provide even more options for thermodynamic models than we can cover in this text. We have included background and comparison on many of the popular thermodynamic models to address this issue.
Computationaladvances are also affecting education. Thus we have significant usage of equations of state throughout the text. We find these computational tools remove much of the drudgery of repetitive calculations, which permits more class time to be spent on the development of theories, molecular perspective, and comparisons of alternative models. We have included FORTRAN, Excel spreadsheets, TI85, and HP48 calculator programs to complement the text. The programs are summarized in the appendices.
- Solutions to cubic equations of state are no longer tedious with the handheld calculators available today for about $100. We provide programs for calculation of thermodynamic properties via the Peng-Robinson equation, vapor pressure programs, Peng-Robinson K-ratios and bubble pressures of mixtures, and van Laar and UNIFAC activity coefficients as well as several other utility programs. Our choice of the HP48 calculator is due to its being one of the first to provide a computer interface for downloading programs from a PC and provide calculator-to-calculator communication, which facilitates distribution of the programs. If all students in the class have access to these engineering calculators, as practiced at the University of Akron, questions on exams can be designed to apply to these programs directly. This obviates the need for traditional methods of reading charts for departure functions and K-ratios and enables treatment of modern methods like equations of state and UNIFAC.
- Spreadsheets have also improved to the point that they are powerful tools for solving engineering problems. We have chosen to develop spreadsheets for Microsoft® Excel because of the widespread availability. Certainly Mathcad®, Mathematica®, and other software could be used, but none has the widespread availability of spreadsheets. We have found the solver within Excel to provide a good tool for solving a wide variety of problems. We provide spreadsheets for thermodynamic properties, phase and reaction equilibria.
- High-level programming is still necessary for more advanced topics. For these applications, we provide compiled programs for thermodynamic properties and phase behavior. For an associating system, such as an alcohol, we provide the ESD equation of state. These programs are menu-driven and do not require knowledge of a computer language.
In a limited number of instances, we provide FORTRAN source code. We provide FORTRAN code because of our own abilities to program faster in FORTRAN, although other languages are finding increasing popularity in the engineering community. We have tried to avoid customization of the code for a specific FORTRAN compiler, which improves portability to other operating platforms but also limits the "bells and whistles" that a specific interface could provide. These programs provide a framework for students and practicing engineers to customize for their own applications.
Fugacity is another property which is difficult to understand. We have tried to focus on the need for a property which is a natural function of T and P, and also stress how it is related to departure functions. There are many ways to calculate fugacities (which provides many trees to block the view of the forest), and we have tried to provide tables and diagrams to show the inter-relations between fugacity coefficients, activity coefficients, ideal gases, ideal solutions, and real solutions.
A distinct feature of this text is its emphasis on molecular physics at the introductory level. Our perspective is that this background must be made available to students in an integrated manner, but it is up to instructors to decide the level of emphasis for the entire spectrum of their students. We have organized this material such that it may be covered as a supplementary reading assignment or as a homework and test assignment. With the latter emphasis, it is possible to formulate a graduate course based on this text.
Throughout the text, we have used text boxes to highlight important statements and equations. Boxed equations are not always final results of derivations. In some cases, the boxes highlight mathematical definitions of important intermediate results that might be useful for homework problems.
We consider the examples to be an integral part of the text, and we use them to illustrate important points. In some cases, derivations and important equations are within an example because the equations are model-specific (e.g., ideal gas). Examples are often cross-referenced and are therefore listed in the table of contents.
There are many marginal notes throughout the text. Where you find an EXCLAMATION POINT icon, it means that an important point is made, or a useful equation has been introduced. Where you find an HP or TI icon, it means that a calculator program is available to assist in calculations. The calculator programs are sometimes not necessary, but extremely helpful. Where you find a DISK icon, it means that an Excel spreadsheet or a compiled program is available. In some cases, the program is simply convenient, but typically you will find that these calculations are tedious without the program. For calculator or PC icons, the program names are given by the icons. See the computer appendix or the readme files for specific program instructions.
We periodically update computer software and the computer appendix. The latest software is available from our website http://www.egr.msu.edu/~lira/thermtxt.htm. We hope you find our approaches helpful in your learning and educational endeavors. We welcome your suggestions for further improvements and enhancements. You may contact us easily at the email addresses below. Unfortunately, we will be unable to personally respond to all comments, although we will try.
Notes to Students
Computer programs facilitate the solution to homework problems, but should not be used to replace an understanding of the material. Always understand exactly which formulas are required before turning to the computer. Before using the computer, we recommend that you know how to solve the problem by hand calculations. If you do not understand the formulas in the spreadsheets it is a good indication that you need to do more studying before using the program so that the structure of the spreadsheet will make sense. When you understand the procedures, it should be obvious which spreadsheet cells will help you to the answer, and which cells are intermediate calculations. It is also helpful to rework example problems from the text using the software.
Acknowledgments
We would like to thank the many people who helped this work find its way to the classroom. We express appreciation to Professors Joan Brennecke, Mike Matthews, Bruce Poling, Ross Taylor, and Mark Thies, who worked with early versions of the text and provided suggestions for improve-ment. We are also greatly indebted to Dave Hart for proofreading an early version. There are many students who suffered through error-prone preliminary versions, and we thank them all for their patience and vision of the common goal of an error-free book. CTL would like to thank Ryoko Yamasaki for her work in typing many parts of the manuscript and problem solutions. CTL also thanks family members Gail, Nicolas, and Adrienne for their patience while the text was prepared, as many family sacrifices helped make this book possible. JRE thanks family members Guliz, Serra, and Eileen for their similar forbearance. We acknowledge Dan Friend and NIST, Boulder for contributions to the steam tables and thermodynamic charts. Lastly, we acknowledge the influences of the many authors of previous thermodynamics texts. We hope we have done justice to this distinguished tradition, while simultaneously bringing deeper insight to a broader audience.
Carl T. Lira, Michigan State University, lira@egr.msu.edu
J. Richard Elliott, University of Akron, dickelliott@uakron.edu"
Table of Contents
Preface xviiAbout the Authors xix
Glossary xxi
Notation xxv
Unit I: First and Second Laws 1
Chapter 1: Basic Concepts 3
1.1 Introduction 5
1.2 The Molecular Nature of Energy, Temperature, and Pressure 6
1.3 The Molecular Nature of Entropy 15
1.4 Basic Concepts 15
1.5 Real Fluids and Tabulated Properties 22
1.6 Summary 33
1.7 Practice Problems 34
1.8 Homework Problems 35
Chapter 2: The Energy Balance 39
2.1 Expansion/Contraction Work 40
2.2 Shaft Work 41
2.3 Work Associated with Flow 41
2.4 Lost Work versus Reversibility 42
2.5 Heat Flow 46
2.6 Path Properties and State Properties 46
2.7 The Closed-System Energy Balance 48
2.8 The Open-System, Steady-State Balance 51
2.9 The Complete Energy Balance 56
2.10 Internal Energy, Enthalpy, and Heat Capacities 57
2.11 Reference States 63
2.12 Kinetic and Potential Energy 66
2.13 Energy Balances for Process Equipment 68
2.14 Strategies for Solving Process Thermodynamics Problems 74
2.15 Closed and Steady-State Open Systems 75
2.16 Unsteady-State Open Systems 80
2.17 Details of Terms in the Energy Balance 85
2.18 Summary 86
2.19 Practice Problems 88
2.20 Homework Problems 90
Chapter 3: Energy Balances for Composite Systems 95
3.1 Heat Engines and Heat Pumps — The Carnot Cycle 96
3.2 Distillation Columns 101
3.3 Introduction to Mixture Properties 105
3.4 Ideal Gas Mixture Properties 106
3.5 Mixture Properties for Ideal Solutions 106
3.6 Energy Balance for Reacting Systems 109
3.7 Reactions in Biological Systems 119
3.8 Summary 121
3.9 Practice Problems 122
3.10 Homework Problems 122
Chapter 4: Entropy 129
4.1 The Concept of Entropy 130
4.2 The Microscopic View of Entropy 132
4.3 The Macroscopic View of Entropy 142
4.4 The Entropy Balance 153
4.5 Internal Reversibility 158
4.6 Entropy Balances for Process Equipment 159
4.7 Turbine, Compressor, and Pump Efficiency 164
4.8 Visualizing Energy and Entropy Changes 165
4.9 Turbine Calculations 166
4.10 Pumps and Compressors 173
4.11 Strategies for Applying the Entropy Balance 175
4.12 Optimum Work and Heat Transfer 177
4.13 The Irreversibility of Biological Life 181
4.14 Unsteady-State Open Systems 182
4.15 The Entropy Balance in Brief 185
4.16 Summary 185
4.17 Practice Problems 187
4.18 Homework Problems 189
Chapter 5: Thermodynamics Of Processes 199
5.1 The Carnot Steam Cycle 199
5.2 The Rankine Cycle 200
5.3 Rankine Modifications 203
5.4 Refrigeration 208
5.5 Liquefaction 212
5.6 Engines 214
5.7 Fluid Flow 214
5.8 Problem-Solving Strategies 214
5.9 Summary 215
5.10 Practice Problems 215
5.11 Homework Problems 216
Unit II: Generalized Analysis of Fluid Properties 223
Chapter 6: Classical Thermodynamics – Generalizations For Any Fluid 225
6.1 The Fundamental Property Relation 226
6.2 Derivative Relations 229
6.3 Advanced Topics 244
6.4 Summary 247
6.5 Practice Problems 248
6.6 Homework Problems 248
Chapter 7: Engineering Equations of State for PVT Properties 251
7.1 Experimental Measurements 252
7.2 Three-Parameter Corresponding States 253
7.3 Generalized Compressibility Factor Charts 256
7.4 The Virial Equation of State 258
7.5 Cubic Equations of State 260
7.6 Solving the Cubic Equation of State for Z 263
7.7 Implications of Real Fluid Behavior 269
7.8 Matching the Critical Point 270
7.9 The Molecular Basis of Equations of State: Concepts and Notation 271
7.10 The Molecular Basis of Equations of State: Molecular Simulation 276
7.11 The Molecular Basis of Equations of State: Analytical Theories 282
7.12 Summary 289
7.13 Practice Problems 290
7.14 Homework Problems 291
Chapter 8: Departure Functions 301
8.1 The Departure Function Pathway 302
8.2 Internal Energy Departure Function 304
8.3 Entropy Departure Function 307
8.4 Other Departure Functions 308
8.5 Summary of Density-Dependent Formulas 308
8.6 Pressure-Dependent Formulas 309
8.7 Implementation of Departure Formulas310
8.8 Reference States 318
8.9 Generalized Charts for the Enthalpy Departure 323
8.10 Summary 323
8.11 Practice Problems 325
8.12 Homework Problems326
Chapter 9: Phase Equilibrium in a Pure Fluid 335
9.1 Criteria for Phase Equilibrium 336
9.2 The Clausius-Clapeyron Equation 337
9.3 Shortcut Estimation of Saturation Properties 339
9.4 Changes in Gibbs Energy with Pressure 342
9.5 Fugacity and Fugacity Coefficient 344
9.6 Fugacity Criteria for Phase Equilibria 346
9.7 Calculation of Fugacity (Gases) 347
9.8 Calculation of Fugacity (Liquids) 348
9.9 Calculation of Fugacity (Solids) 353
9.10 Saturation Conditions from an Equation of State 353
9.11 Stable Roots and Saturation Conditions 359
9.12 Temperature Effects on G and f 361
9.13 Summary 361
9.14 Practice Problems 362
9.15 Homework Problems 363
Unit III: Fluid Phase Equilibria in Mixtures 367
Chapter 10: Introduction to Multicomponent Systems 369
10.1 Introduction to Phase Diagrams 370
10.2 Vapor-Liquid Equilibrium (VLE) Calculations 372
10.3 Binary VLE Using Raoult’s Law 374
10.4 Multicomponent VLE Raoult’s Law Calculations 381
10.5 Emissions and Safety 386
10.6 Relating VLE to Distillation 390
10.7 Nonideal Systems 393
10.8 Concepts for Generalized Phase Equilibria 397
10.9 Mixture Properties for Ideal Gases 401
10.10 Mixture Properties for Ideal Solutions 403
10.11 The Ideal Solution Approximation and Raoult’s Law 404
10.12 Activity Coefficient and Fugacity Coefficient Approaches 405
10.13 Summary 405
10.14 Practice Problems 407
10.15 Homework Problems 407
Chapter 11: An Introduction To Activity Models 411
11.1 Modified Raoult’s Law and Excess Gibbs Energy 412
11.2 Calculations Using Activity Coefficients 416
11.3 Deriving Modified Raoult’s Law 423
11.4 Excess Properties 426
11.5 Modified Raoult’s Law and Excess Gibbs Energy 427
11.6 Redlich-Kister and the Two-Parameter Margules Models 429
11.7 Activity Coefficients at Special Compositions 432
11.8 Preliminary Indications of VLLE 434
11.9 Fitting Activity Models to Multiple Data 435
11.10 Relations for Partial Molar Properties 439
11.11 Distillation and Relative Volatility of Nonideal Solutions 442
11.12 Lewis-Randall Rule and Henry’s Law 443
11.13 Osmotic Pressure 449
11.14 Summary 454
11.15 Practice Problems 455
11.16 Homework Problems 455
Chapter 12: van der Waals Activity Models 465
12.1 The van der Waals Perspective for Mixtures 466
12.2 The van Laar Model 469
12.3 Scatchard-Hildebrand Theory 471
12.4 The Flory-Huggins Model 474
12.5 MOSCED and SSCED Theories 479
12.6 Molecular Perspective and VLE Predictions 483
12.7 Multicomponent Extensions of van der Waals’ Models 486
12.8 Flory-Huggins and van der Waals Theories 491
12.9 Summary 492
12.10 Practice Problems 494
12.11 Homework Problems 495
Chapter 13: Local Composition Activity Models 499
13.1 Local Composition Theory 501
13.2 Wilson’s Equation 505
13.3 NRTL 508
13.4 UNIQUAC 509
13.5 UNIFAC 514
13.6 COSMO-RS Methods 520
13.7 The Molecular Basis of Solution Models 526
13.8 Summary 532
13.9 Important Equations 533
13.10 Practice Problems 533
13.11 Homework Problems 534
Chapter 14: Liquid-Liquid and Solid-Liquid Phase Equilibria 539
14.1 The Onset of Liquid-Liquid Instability 539
14.2 Stability and Excess Gibbs Energy 542
14.3 Binary LLE by Graphing the Gibbs Energy of Mixing 543
14.4 LLE Using Activities 545
14.5 VLLE with Immiscible Components 548
14.6 Binary Phase Diagrams 549
14.7 Plotting Ternary LLE Data 551
14.8 Critical Points in Binary Liquid Mixtures 552
14.9 Numerical Procedures for Binary, Ternary LLE 556
14.10 Solid-Liquid Equilibria 556
14.11 Summary 569
14.12 Practice Problems 570
14.13 Homework Problems 570
Chapter 15: Phase Equilibria in Mixtures by an Equation of State 579
15.1 Mixing Rules for Equations of State 580
15.2 Fugacity and Chemical Potential from an EOS 582
15.3 Differentiation of Mixing Rules 588
15.4 VLE Calculations by an Equation of State 594
15.5 Strategies for Applying VLE Routines 603
15.6 Summary 603
15.7 Practice Problems 604
15.8 Homework Problems 606
Chapter 16: Advanced Phase Diagrams 613
16.1 Phase Behavior Sections of 3D Objects 613
16.2 Classification of Binary Phase Behavior 617
16.3 Residue Curves 630
16.4 Practice Problems 636
16.5 Homework Problems 636
Unit IV: Reaction Equilibria 639
Chapter 17: Reaction Equilibria 641
17.1 Introduction 642
17.2 Reaction Equilibrium Constraint 644
17.3 The Equilibrium Constant 646
17.4 The Standard State Gibbs Energy of Reaction 647
17.5 Effects of Pressure, Inerts, and Feed Ratios 649
17.6 Determining the Spontaneity of Reactions 652
17.7 Temperature Dependence of Ka 652
17.8 Shortcut Estimation of Temperature Effects 655
17.9 Visualizing Multiple Equilibrium Constants 656
17.10 Solving Equilibria for Multiple Reactions 658
17.11 Driving Reactions by Chemical Coupling 662
17.12 Energy Balances for Reactions 664
17.13 Liquid Components in Reactions 667
17.14 Solid Components in Reactions 669
17.15 Rate Perspectives in Reaction Equilibria 671
17.16 Entropy Generation via Reactions 672
17.17 Gibbs Minimization 673
17.18 Reaction Modeling with Limited Data 677
17.19 Simultaneous Reaction and VLE 677
17.20 Summary 683
17.21 Practice Problems 684
17.22 Homework Problems 686
Chapter 18: Electrolyte Solutions 693
18.1 Introduction to Electrolyte Solutions 693
18.2 Colligative Properties 695
18.3 Speciation and the Dissociation Constant 697
18.4 Concentration Scales and Standard States 699
18.5 The Definition of pH 701
18.6 Thermodynamic Network for Electrolyte Equilibria 702
18.7 Perspectives on Speciation 703
18.8 Acids and Bases 704
18.9 Sillèn Diagram Solution Method712
18.10 Applications 723
18.11 Redox Reactions 727
18.12 Biological Reactions 731
18.13 Nonideal Electrolyte Solutions: Background 739
18.14 Overview of Model Development 740
18.15 The Extended Debye-Hückel Activity Model 742
18.16 Gibbs Energies for Electrolytes 743
18.17 Transformed Biological Gibbs Energies and Apparent Equilibrium Constants 745
18.18 Coupled Multireaction and Phase Equilibria 749
18.19 Mean Ionic Activity Coefficients 753
18.20 Extending Activity Calculations to High Concentrations 755
18.21 Summary 755
18.22 Supplement 1: Interconversion of Concentration Scales 757
18.23 Supplement 2: Relation of Apparent Chemical Potential to Species Potentials 758
18.24 Supplement 3: Standard States 759
18.25 Supplement 4: Conversion of Equilibrium Constants 760
18.26 Practice Problems 761
18.27 Homework Problems 761
Chapter 19: Molecular Association and Solvation 767
19.1 Introducing the Chemical Contribution 768
19.2 Equilibrium Criteria 772
19.3 Balance Equations for Binary Systems 775
19.4 Ideal Chemical Theory for Binary Systems 776
19.5 Chemical-Physical Theory 779
19.6 Wertheim’s Theory for Complex Mixtures 782
19.7 Mass Balances for Chain Association 792
19.8 The Chemical Contribution to the Fugacity Coefficient and Compressibility Factor 793
19.9 Wertheim’s Theory of Polymerization 795
19.10 Statistical Associating Fluid Theory (The SAFT Model) 799
19.11 Fitting the Constants for an Associating Equation of State 802
19.12 Summary 804
19.13 Practice Problems 806
19.14 Homework Problems 806
Appendix A: Summary of Computer Programs 811
A.1 Programs for Pure Component Properties 811
A.2 Programs for Mixture Phase Equilibria 812
A.3 Reaction Equilibria 813
A.4 Notes on Excel Spreadsheets 813
A.5 Notes on MATLAB 814
A.6 Disclaimer 815
Appendix B: Mathematics 817
B.1 Important Relations 817
B.2 Solutions to Cubic Equations 822
B.3 The Dirac Delta Function 825
Appendix C: Strategies for Solving VLE Problems 831
C.1 Modified Raoult’s Law Methods 832
C.2 EOS Methods 835
C.3 Activity Coefficient (Gamma-Phi) Methods 838
Appendix D: Models for Process Simulators 839
D.1 Overview 839
D.2 Equations of State 839
D.3 Solution Models 840
D.4 Hybrid Models 840
D.5 Recommended Decision Tree 841
Appendix E: Themodynamic Properties 843
E.1 Thermochemical Data 843
E.2 Latent Heats 846
E.3 Antoine Constants 847
E.4 Henry’s Constant with Water as Solvent 847
E.5 Dielectric Constant for Water 848
E.6 Dissociation Constants of Polyprotic Acids 849
E.7 Standard Reduction Potentials 849
E.8 Biochemical Data 852
E.9 Properties of Water 854
E.10 Pressure-Enthalpy Diagram for Methane 865
E.11 Pressure-Enthalpy Diagram for Propane 866
E.12 Pressure-Enthalpy Diagram for R134a (1,1,1,2-Tetraflouroethane) 867
Index 869
Preface
"No happy phrase of ours is ever quite original with us; there is nothing of our own in it except some slight change born of our temperament, character, environment, teachings and associations."Thank you for your interest in our book. We have developed this book to address ongoing evolutions in applied thermodynamics and computer technology. Molecular perspective is becoming more important in the refinement of thermodynamic models for fluid properties and phase behavior. Molecular simulation is increasingly used for exploring and improving fluid models. While many of these techniques are still outside the scope of this text, these new technologies will be important to practicing engineers in the near future, and an introduction to the molecular perspective is important for this reason. We expect our text to continue to evolve with the chemical engineering field.
Mark Twain
Computer technology has made process simulators commonplace in most undergraduate curriculums and professional work environments. This increase in computational flexibility has moved many of the process calculations from mainframe computers and thermodynamic property experts to the desktop and practicing engineers and students. This increase in computational ability also increases the responsibility of the individuals developing process simulations to choose meaningful models for the components in the system because most simulators provide even more options for thermodynamic models than we can cover in this text. We have included background and comparison on many of the popular thermodynamic models to address this issue.
Computational advances are also affecting education. Thus we have significant usage of equations of state throughout the text. We find these computational tools remove much of the drudgery of repetitive calculations, which permits more class time to be spent on the development of theories, molecular perspective, and comparisons of alternative models. We have included FORTRAN, Excel spreadsheets, TI85, and HP48 calculator programs to complement the text. The programs are summarized in the appendices.
- Solutions to cubic equations of state are no longer tedious with the handheld calculators available today for about $100. We provide programs for calculation of thermodynamic properties via the Peng-Robinson equation, vapor pressure programs, Peng-Robinson K-ratios and bubble pressures of mixtures, and van Laar and UNIFAC activity coefficients as well as several other utility programs. Our choice of the HP48 calculator is due to its being one of the first to provide a computer interface for downloading programs from a PC and provide calculator-to-calculator communication, which facilitates distribution of the programs. If all students in the class have access to these engineering calculators, as practiced at the University of Akron, questions on exams can be designed to apply to these programs directly. This obviates the need for traditional methods of reading charts for departure functions and K-ratios and enables treatment of modern methods like equations of state and UNIFAC.
- Spreadsheets have also improved to the point that they are powerful tools for solving engineering problems. We have chosen to develop spreadsheets for Microsoft® Excel because of the widespread availability. Certainly Mathcad®, Mathematica®, and other software could be used, but none has the widespread availability of spreadsheets. We have found the solver within Excel to provide a good tool for solving a wide variety of problems. We provide spreadsheets for thermodynamic properties, phase and reaction equilibria.
- High-level programming is still necessary for more advanced topics. For these applications, we provide compiled programs for thermodynamic properties and phase behavior. For an associating system, such as an alcohol, we provide the ESD equation of state. These programs are menu-driven and do not require knowledge of a computer language.
In a limited number of instances, we provide FORTRAN source code. We provide FORTRAN code because of our own abilities to program faster in FORTRAN, although other languages are finding increasing popularity in the engineering community. We have tried to avoid customization of the code for a specific FORTRAN compiler, which improves portability to other operating platforms but also limits the "bells and whistles" that a specific interface could provide. These programs provide a framework for students and practicing engineers to customize for their own applications.
Fugacity is another property which is difficult to understand. We have tried to focus on the need for a property which is a natural function of T and P, and also stress how it is related to departure functions. There are many ways to calculate fugacities (which provides many trees to block the view of the forest), and we have tried to provide tables and diagrams to show the inter-relations between fugacity coefficients, activity coefficients, ideal gases, ideal solutions, and real solutions.
A distinct feature of this text is its emphasis on molecular physics at the introductory level. Our perspective is that this background must be made available to students in an integrated manner, but it is up to instructors to decide the level of emphasis for the entire spectrum of their students. We have organized this material such that it may be covered as a supplementary reading assignment or as a homework and test assignment. With the latter emphasis, it is possible to formulate a graduate course based on this text.
Throughout the text, we have used text boxes to highlight important statements and equations. Boxed equations are not always final results of derivations. In some cases, the boxes highlight mathematical definitions of important intermediate results that might be useful for homework problems.
We consider the examples to be an integral part of the text, and we use them to illustrate important points. In some cases, derivations and important equations are within an example because the equations are model-specific (e.g., ideal gas). Examples are often cross-referenced and are therefore listed in the table of contents.
There are many marginal notes throughout the text. Where you find an EXCLAMATION POINT icon, it means that an important point is made, or a useful equation has been introduced. Where you find an HP or TI icon, it means that a calculator program is available to assist in calculations. The calculator programs are sometimes not necessary, but extremely helpful. Where you find a DISK icon, it means that an Excel spreadsheet or a compiled program is available. In some cases, the program is simply convenient, but typically you will find that these calculations are tedious without the program. For calculator or PC icons, the program names are given by the icons. See the computer appendix or the readme files for specific program instructions.
We periodically update computer software and the computer appendix. The latest software is available from our website http://www.egr.msu.edu/~lira/thermtxt.htm. We hope you find our approaches helpful in your learning and educational endeavors. We welcome your suggestions for further improvements and enhancements. You may contact us easily at the email addresses below. Unfortunately, we will be unable to personally respond to all comments, although we will try.
Notes to Students
Computer programs facilitate the solution to homework problems, but should not be used to replace an understanding of the material. Always understand exactly which formulas are required before turning to the computer. Before using the computer, we recommend that you know how to solve the problem by hand calculations. If you do not understand the formulas in the spreadsheets it is a good indication that you need to do more studying before using the program so that the structure of the spreadsheet will make sense. When you understand the procedures, it should be obvious which spreadsheet cells will help you to the answer, and which cells are intermediate calculations. It is also helpful to rework example problems from the text using the software.
Acknowledgments
We would like to thank the many people who helped this work find its way to the classroom. We express appreciation to Professors Joan Brennecke, Mike Matthews, Bruce Poling, Ross Taylor, and Mark Thies, who worked with early versions of the text and provided suggestions for improve-ment. We are also greatly indebted to Dave Hart for proofreading an early version. There are many students who suffered through error-prone preliminary versions, and we thank them all for their patience and vision of the common goal of an error-free book. CTL would like to thank Ryoko Yamasaki for her work in typing many parts of the manuscript and problem solutions. CTL also thanks family members Gail, Nicolas, and Adrienne for their patience while the text was prepared, as many family sacrifices helped make this book possible. JRE thanks family members Guliz, Serra, and Eileen for their similar forbearance. We acknowledge Dan Friend and NIST, Boulder for contributions to the steam tables and thermodynamic charts. Lastly, we acknowledge the influences of the many authors of previous thermodynamics texts. We hope we have done justice to this distinguished tradition, while simultaneously bringing deeper insight to a broader audience.
Carl T. Lira, Michigan State University, lira@egr.msu.edu
J. Richard Elliott, University of Akron, dickelliott@uakron.edu"