* Covers the role of nucleases in biological systems, with focus on understanding their role in causing human diseases.
* Covers the role of nucleases in biological systems, with focus on understanding their role in causing human diseases.
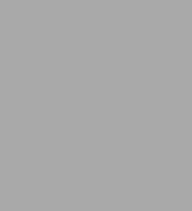
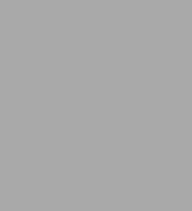
Hardcover
-
SHIP THIS ITEMIn stock. Ships in 6-10 days.PICK UP IN STORE
Your local store may have stock of this item.
Available within 2 business hours
Related collections and offers
Overview
* Covers the role of nucleases in biological systems, with focus on understanding their role in causing human diseases.
Product Details
ISBN-13: | 9780471394617 |
---|---|
Publisher: | Wiley |
Publication date: | 09/06/2002 |
Pages: | 344 |
Product dimensions: | 6.31(w) x 9.48(h) x 0.84(d) |
About the Author
Read an Excerpt
Nucleases
Molecular Biology and ApplicationsBy Nawin C. Mishra
John Wiley & Sons
ISBN: 0-471-39461-0Chapter One
INTRODUCTIONThe genetic blueprint of all organisms consists of nucleic acids. In nature this choice is based on the stability of nucleic acid molecules due to the presence of phosphodiester linkages, which are the strongest among all chemical bonds (Westheimer, 1987). The stability of nucleic acid is a very important feature in maintaining the integrity of the genetic material. However, during the life of an organism the stability of phosphodiester linkages is compromised at times to facilitate certain other important processes such as the removal of damaged DNA and its repair in order to restore the accuracy of the genetic blueprint. Such breakage of phospodiester bonds in DNA chains is also allowed to provide the recombination of genes in a chromosome or the salvage of genetic material in a cell for reutilization of nucleotides or their components or for their final disposal in a cell destined for apoptosis during normal development of a multicellular organism as complex as human. Thus, it is not surprising that all living systems contain a group of enzymes capable of hydrolyzing the phosphodiester linkages present in nucleic acids; these enzymes are called nucleases.
The facts that these phosphodiester linkages are the most stable among all chemical bonds found in biological molecules and that nucleases can hydrolyzesuch phosphodiester linkages make them the most unique of all enzymes. No other groups of enzymes influence the physiology of an organism in such diverse ways as the nucleases. The fact that nucleases catalyze an array of biochemical reactions, all involving just one chemical bond, namely, the phosphodiester linkage, has no parallel in the biochemistry of enzymes. A number of nucleases possess other enzymatic functions in addition to their principal property of catalyzing the hydrolysis of phophodiester linkages. Many nucleases possess other associated catalytic activities such as DNA polymerase, ligase, helicase, or kinase and other functions such as repressor.
Initially, nucleases were considered to play only a degradative role in the salvage pathway of nucleic acid metabolism because of their association with the pancreas (Kunitz, 1940). However, they are now shown to play a multitude of important roles in different aspects of basic genetic mechanisms. These include their participation in (a) the processes of DNA replication, repair, recombination, and mutagenesis (Clark, 1971; Hanawalt et al., 1979; Kornberg and Baker, 1992), (b) the control of gene expression by determining the nature of transcript (Sharp, 1981) and its turnover and (c) transposition and other programmed gene rearrangements (Borst and Greaves, 1987). They are also a part of the host defense mechanism against alien nucleic acid molecules (Luria and Human, 1952). Nucleases play a great role in the mechanisms of immune systems in mammals by controlling the assembling of immunoglobulin genes, their allelic exclusion, and class switching and in the determination of the membrane-bound or secreted forms of the immunoglobulins, leading to immunoglobulin diversity (Sakano et al., 1980; Tonegawa 1983, 1985; Yancopoulos and Alt, 1985, 1988; Yancopoulos et al., 1986) and antigenic variation (Myler et al., 1984; Borst and Cross, 1982). Nucleases also play an important role during programmed cell death in the development of multicellular organisms, including human.
Thus the study of nucleases has been very useful from both the conceptual and technical points of view. The pancreatic ribonuclease was the first enzyme whose entire amino acid sequence was determined (Moore and Stein, 1973; Anfinsen, 1963). The amino acid sequence of the bovine pancreatic ribonuclease is presented in Figure 1.1. Knowledge of the amino acid sequence of the pancreatic ribonuclease was crucial in the confirmation of the idea that the secondary and tertiary structures of a protein are controlled by its primary structure (Anfinsen, 1964). Determination of the structure of the ribonuclease led to the development of an entirely new technology of protein synthesis and protein engineering (Gutte and Merrifield, 1971; Merrifield, 1986; Gutte, 1992). Nucleases have been studied extensively in order to seek answers to the question of the mechanism of enzyme catalysis and polypeptide folding (Anfinsen, 1964; 1973; Tucker et al., 1979; Cotton et al., 1979; Shortle, 1983; Botstein and Shortle, 1985; Kippen et al., 1994). Ribonucleases were used in the complete sequencing of the first tRNA molecule which led to the understanding of its role in the mechanism of genetic coding (Holley, 1965). Use of these nucleases led to the elucidation of the nucleosomal organization of eukaryotic chromosomes (Hewish and Burgoyne, 1973). Above all, the discovery of a new class of nucleases called restriction endonucleases (Smith and Wilcox, 1970) resulted in the development of recombinant DNA technology (Jackson et al., 1972), DNA sequencing methodology (Maxam and Gilbert, 1977; Sanger et al., 1977), and new methods for genetic mapping (Southern, 1975, 1982; Kan and Dozy, 1978; Botstein et al., 1980; White and Lalouel, 1988) and the extensive mapping of human chromosomes (Donis-Keller et al., 1987). Furthermore, the use of restriction endonucleases has been crucial in the development of a new branch of genetics called reverse genetics (Ruddle 1984; Orkin, 1986). The methods of reverse genetics have been very useful in the understanding of the molecular basis of several human diseases-for example, Huntington's chorea, cystic fibrosis, retinoblastoma, arthritis, and many others (White, 1986; Gusella, 1986; Caskey, 1987; Davies, 1991; Mark, 1987; Monaco and Kunkel, 1987; Woron and Thompson, 1988; Martin, 1987; Buxton, 1992)-which may pave the way for the possible alleviation of certain inherited human diseases by gene therapy (Weatherall, 1982; Anderson, 1992; Ailliton, 1985; Nichols, 1988; Mulligan, 1993). All these new methodologies of genetics in which nucleases play critical roles hold great promise for the future of mankind through the development of science, technology, and commerce (Mishra, 1985).
I. HISTORICAL PERSPECTIVES
The enzymatic breakdown of nucleic acid was first observed in the early twentieth century (Araki, 1903). The enzymes involved in this process were named nucleases (Iwanoff, 1903). The fact that nucleases are indeed phosphodiesterases was, however, established much later (Brown and Todd, 1955; Hilmoe et al., 1961; Laskowski, 1967; Westheimer, 1987; Gerlt 1992, 1993). Soon two groups of nucleases designated as ribonuclease (RNase) and deoxyribonuclease (DNase), capable of hydrolyzing ribonucleic acids and deoxyribonucleic acids, respectively, were described by Kunitz (1940, 1950). This specificity of nucleases toward the sugar moiety (i.e., deoxyribose or ribose) of nucleic acid was considered the most important criterion for the classification of nucleases (Kunitz, 1940, 1950). Studies of the properties of crystallized nucleases (Kunitz, 1940, 1950) laid down the foundation for the understanding of the biochemical nature, genetic control, and physiological role of these enzymes. Early studies of nucleases and their chemical modifications provide an excellent model for understanding the nature of the biochemical reaction mechanisms and the role of catalytic sites that facilitate substrate binding. These conclusions were later confirmed by x-ray crystallography. Analysis of the crystal structures of a number of nucleases such as Escherichia coli DNA polymerase I, RNaseH, endonuclease III, topoisomerase I, Hin invertase, [T.sub.4] endonuclease DNaseI, a number of recombinases and structure-selective nucleases pancreatic RNases, and several other RNases has provided insight into the structure and function of enzyme proteins and their specificity of action (Joyce and Steitz, 1987; Suck et al., 1984; Nakamura et al., 1991; Morikawa et al., 1992; Sevcik et al., 1990; Lima et al., 1994).
The study of nucleases, particularly the mechanistic view of the interaction of their active sites with their substrates, has provided a better understanding of the mechanisms of enzyme catalysis (Gerlt, 1992, 1993). Nucleases must possess the ability not only to recognize and bind with a DNA sequence, but also to hydrolyze it. Nucleases differ from other DNA recognizing and binding proteins such as transcription factors; the latter usually possess leucine zipper or zinc finger or helix-turn-helix motifs. Nucleases lack such motifs and therefore must recognize and bind with DNA by other methods. Certain restriction endonucleases (see Chapter 4) may undergo mutation to produce the mutant forms of the enzyme which can recognize a DNA sequence and bind with it but are unable to hydrolyze it.
II. PROTEIN, RNA, DNA, AND OTHER MOLECULES AS NUCLEASES
Enzymes are biocatalysts that facilitate chemical reactions in biological systems. Enzymes are traditionally known to be proteins. However, proteins are no longer the only molecules that have a catalytic function. In recent years a number of RNA and DNA molecules have been shown to possess such functions. Thus, it is no surprise that a number of nucleases are RNA or DNA even though a majority of nucleases are made up of proteins. In addition to proteins and RNAs or DNAs, a number of other smaller molecules (such as bleomycin and phenanthroline and others) have been shown to possess nucleolytic property. It is proposed here that the term enzyme or proteinzyme should be used to indicate all biocatalysts that are proteins. This nomenclature would be consistent with the fact that a majority of molecules with biocatalytic function are proteins. The RNA and DNA molecules with catalytic activities have been designated as ribozyme or RNAzyme (Kruger et al., 1982) and deoxyribozyme or DNAzyme (Breaker and Joyce, 1994; Breaker, 2000; Wilson and Szostak, 1999) respectively. Certain small molecules and chemical reagents with enzyme-like catalytic functions are called chemzymes (Corey and Reichard, 1989; Waldrop, 1989). The occurrence and the role of DNAzymes and chemzymes in biological systems remain to be established. An understanding of the divergent nature of the biocatalysts has made it possible to design synthetic or semisynthetic nucleases that can be targeted to a specific nucleic acid sequence. These nucleases are called artificial or designer or chemical nucleases (Sigman and Chen, 1990). The ability to design such nucleases may revolutionize the field of genome mapping (Ebright et al., 1990; Oakley and Dervan, 1990). Nucleases as protein enzymes are described in the different chapters of this book. The nature and role of ribozymes, deoxyribozymes, and certain chemzymes as nucleases are discussed in Chapter 9. All protein enzymes and ribozymes are encoded by genes or DNA molecules; therefore the DNA molecules are their genotypes and they represent phenotypes, whereas in the case of DNAzyme, both the genotypes and phenotypes are represented by the same molecule.
III. NATURE OF ENZYMATIC REACTIONS CATALYZED BY NUCLEASES
Nucleases are, in essence, phosphoesterases that hydrolyze the internucleotide linkage in a nucleic acid molecule. There are different kinds of phosphoesterases. Phosphomonoesterases do not act on internucleotide linkage but cleave the terminal phosphate from a nucleotide chain. Among the three classes of phosphodiesterases, the first group of enzymes acts on phosphodiester bonds not involving internucleotide linkage. The enzymes responsible for the breakdown of cAMP and cGMP are examples of such phosphodiesterases. The second group of enzymes acts on different types of phosphodiester bonds, including internucleotide linkages; the snake venom phosphodiesterases belong to this group of enzymes. The third group specifically acts on internucleotide phosphodiester linkage(s); protein and ribozyme nucleases belong to this group of phosphodiesterases. Both protein and ribozyme nucleases act by hydrolysis of phosphodiester linkages in the nucleic acid chain. This, however, is not true of the chemzyme nucleases; they cause cleavage by disruption of the sugar moiety via oxidation or by alkylation of bases (Sigman and Chen, 1990).
Most enzymatic reactions are reversible in nature. Both the synthesis and degradation of micromolecules are usually carried out by the same enzymes. However, the degradation and biosynthesis of macromolecules in all biological systems are carried out by distinct sets of enzymes (Kornberg, 1974). Nucleases that cleave internucleotide linkages during the degradation of nucleotides cannot reform the internucleotide linkage leading to the biosynthesis of nucleic acid molecules. Instead, the internucleotide linkages are either (a) formed by DNA or RNA polymerases during the synthesis of specific nucleic acid molecules or (b) joined by the enzyme ligase. However, there are exceptions to this rule. A number of DNA polymerases carry nuclease activity in different parts of the same polypeptide. Also, a class of nucleases called topoisomerases has been shown to combine both properties; that is, they can hydrolyze the internucleotide linkages transiently and then rejoin them as discussed in Chapter 6. The action of different enzymes that act on nucleic acids are illustrated in Figure 1.2. Nucleases and phosphodiesterases cleave the bond between phosphorus and oxygen in the internucleotide linkage of nucleic acid (Hilmoe et al., 1961) as shown in Figure 1.3.
Protein nucleases are characterized by their processive or distributive mode of action. A processive enzyme remains engaged with the substrate molecules, resulting in the hydrolysis of successive internucleotide linkages before it leaves to act on another nucleic acid molecule. In contrast, a distributive nuclease will usually hydrolyze only a few internucleotide linkages before it falls off the substrate molecule and will then be free to engage with the same or another nucleic acid molecule.
IV. CLASSIFICATION
Kunitz (1940) classified nucleases into two major groups, ribonucleases and deoxyribonucleases, based on the specificity of their nucleolytic attack toward the sugar moiety of the different nucleic acid molecules. Even though snake venom phosphodiesterase hydrolyzed both ribonucleotides and deoxyribonucleotides (Schmidt, 1955), the classification of nucleases into DNase and RNase by Kunitz was considered valid at that time. This was partly because of the nonspecific nature of snake venom phosphodiesterase that could attack phosphodiester linkages other than those found in nucleic acid. The classification of nucleases into DNase and RNase, however, appeared somewhat inappropriate with the discovery of micrococcal nuclease and snake venom phosphodiesterases that attacked both DNA and RNA (Laskowski, 1982).
Continues...
Excerpted from Nucleases by Nawin C. Mishra Excerpted by permission.
All rights reserved. No part of this excerpt may be reproduced or reprinted without permission in writing from the publisher.
Excerpts are provided by Dial-A-Book Inc. solely for the personal use of visitors to this web site.
Table of Contents
Preface.List of Nobel Prize Winners for Their Research Work with Nucleases.
About the Author.
1. Introduction.
I. Historical Perspectives.
II. Protein, RNA, DNA, and Other Molecules as Nucleases.
III. Nature of Enzymatic Reactions Catalyzed by Nucleases.
IV. Classification.
A. Nature of Substrates.
B. Mode of Attack.
C. Site-Specificity and Structure-Selectivity.
V. Methods for the Study of Nucleases.
A. Methods for the Assay of the Enzymatic Activity.
B. Methods for the Study and Characterization of Nucleases.
VI. Genetics of Nucleases and Biological Roles.
VII. Applications of Nucleases.
2. Ribonuclease.
I. General Ribonucleases.
A. Microbial Ribonucleases.
1. RNaseT1.
2. RNaseT2.
B. Mammalian Ribonucleases.
1. Bovine Pancreatic Rnase.
2. RNaseA.
3. Human Pancreatic Ribonuclease (HPR).
4. Human Nonsecretory Ribonuclease (HNSR).
5. Human Major Basic Protein (MBP), Eosinophil Cationic Protein (ECP) and Eosinophil-Derived Neurotoxin (EDN).
6. Angiogenin.
7. Interferon-Induced Mammalian Ribonuclease.
8. Human RNase with a Possible Role in Tumor Suppression.
C. Plant Ribonucleases.
D. Evolution of Ribonucleases.
II. Ribonucleases Involved in RNA Processing (Trimming, Splicing, and Editing).
A. RNaseIII and RNaseIII-Like Enzymes.
B. RNaseP.
C. RNaseE.
D. RNaseM5.
E. RNaseD.
F. Eukaryotic RNA-Splicing Enzymes.
1. Yeast tRNA Splicing Endonuclease.
III. Ribonuclease H.
A. E. coli RNaseH.
B. Retroviral Reverse Transcriptase RNaseH.
C. Yeast RNaseH.
D. Human RNaseH.
E. Other Eukaryotic RNaseH.
F. Biological Function of RNaseH.
IV. Proofreading Activity of RNA Polymerase.
3. Deoxyribonuclease.
I. Classification of Enzymes.
A. Deoxyribonucleases.
B. Endonucleases
C. Exonuclease.
II. Properties of Enzymes from Different Organisms.
A. Bacterial Enzymes.
1. Exonuclease I.
2. Exonuclease II.
3. Exonuclease III.
4. Application of the Enzyme Exonuclease III.
5. Exonucleases IVA and IVB.
6. Exonuclease V (RecBCD Enzyme).
7. RecBCD (Exo V) from Other Organisms.
8. Exonuclease VI.
9. Exonuclease VII.
10. Exonucleases Associated with DNA Polymerases.
11. Exonuclease VIII.
B. Endonucleases.
1. Bacterial Enzymes.
2. Mammalian Deoxyribonuclease.
4. Restriction Endonucleases.
I. Occurrence, Classification, and Their General Properties.
A. Different Restriction Endonucleases and Their Properties.
II. Type I Restriction Endonucleases.
A. Purification and General Properties.
B. Recognition Sequences and Nature of Substrate.
C. Different Kinds of Type II Restriction Endonucleases.
D. Genetics.
E. Cleavage Mechanism.
III. Type II Restriction Endonucleases.
A. Enzyme Purification and Assay.
B. General Properties of the Enzyme.
C. Crystal Structure of the Restriction Endonucleases.
D. Reaction Conditions and Enzyme Specificity.
E. Nature of Substrate.
1. Synthetic Oligonucleotides.
2. DNA with Base Analogs.
3. Methylated DNA.
4. Single-Stranded DNA.
5. DNA-RNA Hybrids as Substrate.
F. Inhibition of Restriction Endonucleases.
G. Restriction Endonuclease Genes.
IV. Type III Restriction Endonucleases.
V. Evolutionary Significance and Biological Role.
VI. Application of Restriction Nucleases.
VII. General Tips for Beginners or the First-Time Users of Restriction Enzymes.
5. Damage-Specific Nucleases.
I. Classification and Assay .
A. AP Endonucleases.
B. Enzymes that Directly Attack Phosphodiester Linkages in the Damaged DNA Region.
C. Assay.
II. Properties of Two Groups of Enzymes from Different Organisms.
A. AP Endonucleases.
1. AP Endonucleases Associated with DNA Glycosylase Activity.
2. M. luteus Enzyme.
3. E. coli Endonuclease III.
B. AP Endonuclease Associated with Other Enzyme Activities.
1. E. coli Exonuclease III AP-Endonuclease Activity.
C. AP Endonucleases.
1. E. coli AP Endonucleases.
2. Fungal Apurinic Endonuclease.
3. Drosophila AP Endonucleases.
4. Human AP Endonucleases.
5. Plant AP Endonuclease.
D. Direct-Acting Enzymes.
1. E. coli UV Endonuclease.
2. Human Excision Nuclease.
6. Topoisomerases.
I. Choreography and Topology of DNA.
II. Enzyme Assay.
A. Electron Microscopy.
B. Sedimentation Methods.
C. Agarose Gel Electrophoresis.
III. Properties of Enzymes from Different Groups of Organisms.
A. Prokaryotic Topoisomerases.
1. Prokaryotic Topoisomerase I.
2. Prokaryotic Topoisomerase II.
3. Properties of Gyrase.
4. Other Activities of Gyrase.
5. Prokaryotic Topoisomerase III.
B. Eukaryotic Topoisomerases.
1. Eukaryotic Topoisomerase I.
2. Eukaryotic Topoisomerase II.
C. Mitochondrial Topoisomerase.
D. Viral Topoisomerases.
IV. Genetics and Biological Role.
A. Prokaryotic Topoisomerase Mutants.
1. Topoisomerase I.
2. Topoisomerase II.
B. Eukaryotic Topoisomerase Mutants.
1. Topoisomerase I Mutants of Yeast.
2. Topoisomerase II Mutants of Yeast.
3. Topoisomerase Mutants of Higher Eukaryotes.
7. Recombinases.
I. General Description and Classification.
A. General Recombinase.
B. Site-Specific Recombinase.
1. Prokaryotic.
2. Eukaryotic.
C. Transpositional Recombinase.
D. RNA Recombinase.
II. Properties of Different Recombinases.
A. General Recombinase.
1. Initiase.
2. X-Solvase.
3. Correctase.
B. Site-Specific Recombinase.
C. Prokaryotic Site-Specific Recombinase.
1. Integrase.
2. Invertase.
3. Resolvase.
D. Eukaryotic Site-Specific Recombinase.
1. Eukaryotic Site-Specific Recombinase.
2. ''Homing'' Nuclease (Intron Coded Nuclease).
3. Viral Integrase.
E. Transpositional Recombinase.
1. Prokaryotic Transposases.
2. Eukaryotic Transposase.
3. Retrotransposable Elements and Retrotransposases.
F. Control of Recombinases.
G. RNA Recombinase.
8. Sugar-NonSpecific Nucleases.
I. General Description, Classification, and Methods of Assay.
II. Properties of Enzymes from Different Groups of Organisms.
A. Microbial Nucleases.
1. Neurospora crassa Endonuclease.
2. S1 Nuclease.
3. Yeast Nucleases.
4. Micrococcal Nuclease.
5. Bal-31 Nucleases.
B. Animal Nucleases.
1. Spleen Exonucleases.
2. Snake Venom Exonuclease.
C. Plant Nucleases.
1. Mung Bean Endonuclease.
2. Other Plant Nucleases.
D. Parasitic Protozoan Nuclease.
9. Nonprotein Nucleases.
I. Ribozymes.
A. RNaseP.
1. Protein Component of RNaseP.
B. Introns as Ribozymes.
1. Group I Intron Ribozymes.
2. Mechanism of Catalysis by Group I Intron Ribozyme.
3. Assay of Ribozyme Activity of Intron RNA or Other RNA.
C. Group II Intron Ribozymes.
D. Splicosomal snRNA Ribozyme.
1. Proteins that Facilitate the Ribozyme Activity of RNA Nucleases.
E. Maturase.
F. Hammerhead RNA as Ribozyme.
G. Cis- and Trans-Acting Ribozyme Endonuclease.
II. DNAzymes.
III. Chemzymes.
A. Chemicals and Metal Ligand Complexes as Nucleases.
1. Piperidine.
2. Hydrogen Peroxide.
3. DNA Intercalating Agents.
4. Phenanthroline.
5. Factors Controlling the DNA Cleavage by Chemzymes.
B. Peptides.
IV. Designer Nuclease.
10. Molecules that Interact with Nucleases.
I. Inhibitors.
A. Proteins as Nuclease Inhibitors.
1. DNase Inhibitor-Protein.
2. RNase Inhibitor-Protein. B. RNA as Nuclease Inhibitors.
C. Other Molecules that Act as Nuclease Inhibitors.
II. Proteins that Interfere with the Activity of Nuclease by Interacting with the Substrate (Nucleic Acids).
III. DNA Sequences that Interact with Nucleases.
A. Chi-Like Elements in Eukaryotes.
IV. Other Inhibitor Molecules.
V. Proteins that Interact with DNA or Nuclease to Orchestrate the Activity of Nucleases.
11. Biological Function of Nucleases.
I. Replication.
A. Three Steps in DNA Replication.
1. Initiation.
2. Elongation.
3. Termination.
B. Role of Viral Nuclease in the Degradation of Host DNA.
C. Involvement of Nuclease During the Separation of Daughter Helices at the End of Replication.
D. Involvement of Nucleases in the Rolling Circle Mechanism of DNA Replication.
E. Involvement of Nuclease in the Replication of Linear DNA.
F. Involvement of Nuclease in the Replication of Chromosome in Eukaryotes.
II. DNA Repair.
A. Baseless Sites.
B. Sites with Altered Base or Incorrect Base.
C. Cross-Linking and Other Damages.
D. DNA Repair Mechanisms.
E. Excision Repair.
F. Bypass Repair Pathways.
G. Recombinational Repair Pathway.
H. Inducible and Error-Prone Repair Pathway.
I. Mismatch Repair.
J. Mismatch Repair in Mammalian Cells.
K. Incision of Damaged DNA is a Complex Process Involving Several Proteins.
L. Excision Repair Mutants of Neurospora.
M. Excision Repair Mutants of Yeast.
N. Excision Repair Mutants of Drosophila.
O. Excision Repair Mutants of Mammalian Cells.
III. Recombination.
A. Different Kinds of Genetic Recombination.
B. Recombination Mechanisms and Nucleases.
C. Gene Conversion and Postmeiotic Segregation.
D. In Vitro Recombination System.
E. Fungal Recombination Nucleases.
F. Mismatch Repairs During Recombination.
G. Recombination Pathways.
1. RecBCD Pathway.
2. RecFJ Pathway.
3. RecE Pathway.
4. Red Pathway.
H. Recombinational Control of Gene Expression.
I. Role of Recombinase in Mammalian Antibody Diversity, Allelic Exclusion, and Class Switch.
J. T-Cell Surface Receptor.
K. Application of Recombinases: Engineered Expression of Genes.
IV. DNA Transfection or Transformation.
V. Mutation.
VI. DNA Supercoiling and Maintenance of Chromosome Structure.
VII. Transcription.
VIII. RNA Processing.
A. RNA Trimming.
B. RNA Splicing.
C. RNA Editing.
IX. Control of Translation.
X. Viral Maturation and Encapsidation.
XI. Nuclease in Defense Mechanism.
XII. Apoptosis and Nucleic Acid Salvage.
12. Nucleases and Human Diseases: Basis for Application.
I. Involvement of Nucleases in Human Disease.
A. Xeroderma Pigmentosum.
B. Ataxia Telangiectasia.
C. Cockayne Syndrome.
D. Cancer.
E. Aging-Werner Syndrome.
F. Immunological Diseases.
G. Nucleases and Neurological Disorders.
H. Human Diseases Involving Defective Protein Folding.
I. Other Human Diseases.
II. Reverse Genetics, Human Diseases, and Nucleases.
III. Use of Nucleases in Control of Human Disease.
13. Nucleases as Tools.
I. Nature of ''Transforming Principle'' as DNA.
II. Isolation of DNA and RNA.
III. Nearest-Neighborhood Analysis.
IV. Isolation of a Gene.
V. Uniparental Transmission During Cytoplasmic Inheritance.
VI. Physical Mapping of DNA.
VII. Use of Nuclease in the Development of Recombinant DNA Technology and the Molecular Cloning of a Gene.
VIII. Construction of an Artificial Chromosome.
IX. New Method for Mapping Eukaryotic Chromosomes.
A. Chromosome Walking (Overlap Hybridization).
B. Role of Nucleases in Transposon Mobility.
X. Use of Nuclease in the Physical Mapping of a Mutational Site.
XI. Biological Activity of a DNA Segment.
A. Use of Nucleases in the Identification of the Function of a DNA Segment via Transformation Experiments.
B. Use of Nucleases in the Deletion Mapping of Biological Activity.
C. Use of Nuclease in Identification of the Function of DNA Segment via Marker Rescue Method.
XII. Organization of Eukaryotic Chromosomes.
XIII. Distinction Between Active and Inactive Genes: The Relation Between Activity of a Gene and a Nuclease-Sensitive Site.
XIV. DNase Footprinting.
XV. Construction of Mutants: Site-Specific Mutation and Protein Engineering.
XVI. Nucleases in Directed Mutagenesis.
XVII. Nick Translation and Labeling of DNA with High-Specificity Radioactivity.
XVIII. Role of Nucleases in PCR.
A. Proofreading by Nuclease During PCR Amplification.
B. Application of 50 Nuclease in PCR Assay for Rapid Detection of a Known Gene in a DNA Sample(s).
C. Application of Nucleases in SNP-Genotyping and Pharmacogenetics.
XIX. Gene Knockout.
XX. RNase Protection Assay.
XXI. Use of Nucleases in Forensic Science.
XXII. Human and Other Genome Projects.
14. Application of Nucleases in Biotechnology, Medicine, Industry, and Environments.
I. Construction of Recombinant DNA and Molecular Cloning of Genes.
II. Biotechnology of Microorganisms, Plants, Animals and Marine Organisms Based on Recombinant DNA Technology.
III. Application in Medicine.
A. Role of Nucleases in Predictive, Preventive, and Curative Medicine.
B. Drug Designs.
C. Antisense Strategy.
IV. Nuclease Therapeutics and Therapeutic Targets.
A. DNaseI and DNAzyme-Based Therapeutics.
B. RNaseA and Ribozyme-Based Therapeutics.
C. Gene Therapy and Enhancement Therapy.
D. Gene Silencing.
E. RNaseL and Interferon-Mediated Control of Viral Infection and treatment of Cancer.
F. Recombinase-Mediated Control of Gene Expression.
G. Poisoning of Topoisomerase-DNA Intermediates.
V. Application in Forensics.
VI. Application in Industry: Production of Flavor Enhancer of Food and Beverage.
VII. Application in Environmental Problems.
A. Bioremediation.
B. Detection of Microbial Pathogens to Prevent Bioterrorism by 5' Nuclease in PCR Assay.
15. Nucleases and Evolution.
I. Ribozyme as Evidence for the Early World of RNA.
II. Chemzyme, Ribozyme, and Proteinzyme.
III. The Role of Recombinase in Evolution.
A. Present-Day Selfish DNA-Possible Origin From Transposon.
IV. Nucleases and Control of DNA Transactions and Their Roles in Evolution.
V. Role of Nucleases in Directed Mutagenesis: Adaptive Mutation an SOS Response.
VI. Nucleases as Multifunctional Molecules.
VII. DNA Sequence Analysis, Crystal Structure, and Bioinformatics.
VIII. Possible Horizontal Transmission of Nuclease Gene and Intron.
IX. Conclusions and Our Future in the World of Nucleases.
References.
Index.