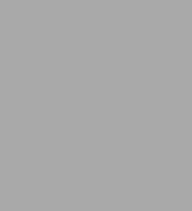
Olfaction and the Brain
382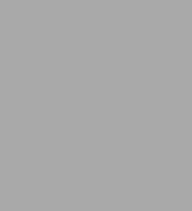
Olfaction and the Brain
382Hardcover
-
SHIP THIS ITEMIn stock. Ships in 3-7 days. Typically arrives in 3 weeks.PICK UP IN STORE
Your local store may have stock of this item.
Available within 2 business hours
Related collections and offers
Overview
Product Details
ISBN-13: | 9780521849227 |
---|---|
Publisher: | Cambridge University Press |
Publication date: | 10/19/2006 |
Pages: | 382 |
Product dimensions: | 7.01(w) x 9.96(h) x 0.87(d) |
About the Author
Warrick Brewer is currently an Associate Professor in the University of Melbourne Department of Psychiatry located at ORYGEN Research Centre, Parkville, where he practises as a Senior Neuropsychologist in the Neuropsychology Clinic.
Nobel Prize winner. See http://nobelprize.org/medicine/laureates/1996/doherty-autobio.html. Peter Doherty was awarded the Nobel Prize in 1996 for his role in discoveries surrounding the specificity of cell-mediated immune defence. http://www.mdhs.unimelb.edu.au/aboutus/staff/
Read an Excerpt
Olfaction and the Brain
Cambridge University Press
978-0-521-84922-7 - Olfaction and the Brain - by Warrick Brewer
Excerpt
Introduction
The olfactory system comprises a sensory organ (the olfactory epithelium) and specific olfactory brain regions, the first of which is the olfactory bulb. The perception of odours poses interesting and different problems for the nervous system – problems unique to the odorous world. The first of these is that there is no single dimension that relates stimulus to sensation. Vision and hearing are stimulated by predictable variations in frequencies of light and sound; touch by variations in frequencies of pressure on the skin. Odorant molecules have no obvious connections with each other except that they are odorous – that is, they evoke sensations in the olfactory system. The second unique attribute of the olfactory system is that there seems to be no limit to the number of odorous molecules that can be detected and described. Vision, hearing and touch all operate within limited spectra of light, sound and pressure, predictable spectra to which the systems have evolved. Odorous molecules are mainly limited to molecules of 200 to 400 mW but within that range, there are essentially an infinite number of odorous molecules. The molecular structures are highly variable and no individual or group of individuals has been exposed to all of the range, or possibly even the majority of the range.
How, then, could a system evolve to detect and respond to such an open ended set of stimuli? The immune system has a similar task and has solved it by using a variable rearrangement of its genetic code to generate protein receptors of huge range. The olfactory system has solved this problem by generating a huge number of individual receptor genes. Of the approximately 30,000 genes in the mouse genome, more than 1000 are for olfactory receptors (Buck & Axel, 1991). It is a source of wonder that one-thirtieth of the genome is devoted to detecting odours. Interestingly, humans have about 900 olfactory receptor gene sequences but 63% are interrupted with sequences so as to render them non-coding, so-called “pseudogenes” (Glusman et al., 2001); although even humans, with their reduced dependence on smell, have about 300 active olfactory receptor genes (Glusman et al., 2001). This begs the question; what is it about the stimulus that requires such a huge investment of genes? The visual system needs only three genes to detect the colour spectrum. The auditory system just requires a specialised physical structure – the cochlea, which is constructed via genes used for multiple other roles in development. The olfactory system requires one-thirtieth of the genome. An intriguing aspect of the olfactory system is that these odorant receptor genes are involved both in odorant detection as well as in establishing the basic anatomy of the olfactory system that allows that detection.
The first part of this chapter provides an overview of the anatomy of the primary olfactory system, the olfactory mucosa and olfactory bulb, and how this contributes to our current understandings of how the chemical properties of odorant molecules are encoded into neural activity. The second part of this review covers the consequences of this neural activity and how it defines the regions of the human brain involved in olfactory perception.
The olfactory epithelium
The olfactory mucosa is the region of the nasal cavity that is specialised for the detection of odorants. It comprises the olfactory epithelium and its underlying lamina propria. The olfactory mucosa is nominally located in the superior and posterior part of the nasal cavity, close to the cribriform plate through which the olfactory nerves project to find the olfactory bulb – the initial olfactory region of the central nervous system (Figure 1.1). In adult humans the olfactory mucosa is not always contiguous and can be found more anteriorly and inferiorly on the nasal septum and lateral wall (Féron et al., 1998; Leopold et al., 2000). Unusually, the olfactory epithelium undergoes a continual process of neurogenesis in which new neurons are continually generated throughout adult life, and this may explain the discontinuity and spread of the olfactory mucosa (Féron et al., 1998). The advantages of utilising this process for mapping neural development more generally are expanded in Chapter 5.
The axons of the sensory neurons leave the olfactory epithelium and gather in bundles within the lamina propria to course superiorly and posteriorly. As they do, they gather into larger fascicles that form the fila olfactoria that traverse the skull base through the many openings of the cribriform plate to enter the olfactory bulb. In the olfactory bulb, the axons defasciculate and enter specialised structures, glomeruli, within which the sensory axons synapse with the mitral cells of the olfactory bulb.
Within the olfactory epithelium, the olfactory sensory neurons express just one of the many odorant receptor genes (Buck & Axel, 1991). Cells expressing each gene are distributed seemingly “randomly” within the olfactory epithelium, subject only to subgroups of these cells being confined to several broad regions within the nasal cavity (Ressler et al., 1993). Surprisingly, when these sensory neurons send their axons to the olfactory bulbs, the glomeruli to which they are restricted are confined to a single glomerulus on either side of the olfactory bulb (Mombaerts et al., 1996). Thus in mice there are approximately 1800 glomeruli (Royet et al., 1988) and approximately 1000 receptor genes (Buck & Axel, 1991). Recent experiments indicate that each glomerulus is innervated by sensory neurons expressing only one receptor gene (Mombaerts et al., 1996). The identity of the receptor gene borne by each sensory neuron is involved in targeting its axon to a specific glomerulus, although the receptor gene is necessary but not sufficient to address the axon to a particular glomerulus.
Olfactory sensory neurons each respond to many different odorants (Duchamp-Viret et al., 1999) even though each is thought to express only a single odorant receptor gene. Thus each odorant receptor protein can apparently detect some aspects of a variety of different odorant molecules and each odorant can interact with multiple receptors (Araneda et al., 2004). This heterogeneity of response continues to provide a conundrum for understanding the relation between odorant molecular properties and the odorant-binding domain within the receptor molecule.
The olfactory bulb
The cellular structure of the olfactory bulb is well established (Mori, 1987). Incoming sensory information passes to the mitral and tufted cells that provide the output to the higher olfactory centres (Figure 1.1). This output is heavily modulated by the interneurons present at several anatomical and processing levels within the olfactory bulb. Around the glomeruli are several types of interneurons, many of which are dopaminergic, whose axons and dendrites form part of the complex neuropil within the glomeruli. Deep in the olfactory bulb are the granule cells, which are without axons, but whose dendrites connect with mitral cell dendrites in more superficial layers, modulating their activity via complementary pairs of synapses from granule cell dendrites to mitral and tufted cells, and in the reverse direction, the so-called “dendrodendritic synapses”.
The physiology of the olfactory bulb is dominated by the spatial nature of the input. Specifically, because of the spatial patterning of the sensory axons expressing different odorant receptor genes, odorants presented in the nose stimulate different patches of glomeruli on the surface of the olfactory bulbs (Figure 1.2). Because each mitral cell has a dendrite in only a single glomerulus, the activity of the mitral cells is spatially distributed such that odorants are represented in the olfactory bulb by a distributed pattern of mitral cell activity (Leon & Johnson, 2003; Lowe, 2003; Mori et al., 1999). The nature of interneuron connectivity then leads to a sharpening of the response of the mitral cell both in time and space, with the effect of narrowing the responses of the mitral cells to a smaller number of odorant molecules compared to the sensory neurons (Lowe, 2003; Mori et al., 1999). Similarly the mitral cell response is narrow in time as well (Lowe, 2003; Mori et al., 1999).
A notable feature of olfactory bulb anatomy is the convergence of feedback from higher centres whose axons project onto the interneurons at the granule and periglomerular levels. Combined with the observation that cellular models of memory are evident in the activity of olfactory bulb neurons (Wilson et al., 2004), these features suggest that the olfactory bulb is not merely a passive conduit for sensory information, modulated and sharpened as it is by interneuron activity. There is ample evidence that the olfactory bulb physiology is modulated by prior exposure and experience with odorants (McLean et al., 1999) and evidence for feedback superimposed on the olfactory bulb (McLean & Harley, 2004). It is thus possible that the olfactory bulb acts as a filter of some sort that matches expected patterns of activity associated with food, mates or predators, for example, with the pattern of sensory nerve activity.
What the nose tells the brain
Any hypothesis about the encoding of odorant molecules must reconcile several aspects of human perception. The first is that “odour” does not refer to any obvious specific molecular feature of the odorant. For example, all odorants containing sulphur smell are “sulphurous” but they do not smell the same. This illustrates the second aspect, namely that there seems to be a huge variety of perceptual experiences of “odour” as there are a huge number of odorants. The third aspect is that “odour” is a holistic experience, such that complex mixtures such as coffee have a single percept, as do single odorant molecules. Humans are relatively poor at discriminating the components of mixtures and cannot distinguish more than three or four separate components (Laing & Francis, 1989). A fourth aspect is that, for most odorants, their identification does not change with concentration: there is perceptual constancy with increasing concentration.
The first evidence proposed for a mechanism of encoding odorants was that different odorants were encoded in spatial patterns of activity in the olfactory bulb (Kauer & White, 2001; Leon & Johnson, 2003). This hypothesis has proved to be prescient, although it has taken many years and the advent of new technologies to provide a satisfactory mechanism to explain these observations. Initial experiments emphasised the spatial differences in activity in the olfactory epithelium, but the apparent complexity in the connectivity between the epithelium and the bulb remained an obstacle to understanding. When the true nature of the connectivity was revealed in the expression of olfactory receptor genes, the focus turned to the glomeruli of the olfactory bulb as the originators of a “spatial code” (Mombaerts et al., 1996; Mori et al., 1999; Ressler et al., 1993).
There are many ways to examine the spatial nature of responses in the olfactory glomeruli. The one that has allowed the most extensive and thorough examination is the method of uptake of 2-deoxyglucose, a molecule accumulated by active neurons that can be revealed by autoradiography in histological sections. After a thorough series of investigations in which the mapping of active glomeruli was correlated with systematic variation in odorant stimuli, several features of a “spatial code for odour” were discerned (reviewed in Leon & Johnson (2003)). It is apparent that the surface of the olfactory bulb is composed of groups of glomeruli, called “modules”, that appear to encode molecular functional groups (Figure 1.3). Thus, for example, all ketones would activate glomeruli in the same modules, which would be spatially separate from the module activated by acids or alcohols (Leon & Johnson, 2003). Molecules of similar structure, such as organic acids with increasing carbon chain lengths, would activate neighbouring glomeruli, with the focus of activity moving systematically across the surface of the olfactory bulb. These observations suggest that olfactory bulb activity is “chemotopically” organised, its role being to parse the odorant signal into a set of activities representing functional groups, carbon chain length and other structural features (Leon & Johnson, 2003).
These observations at the level of the glomerulus are reinforced by observations of the responses of mitral cells after systematic analyses of their responses to odorants within molecular series reviewed by Mori et al. [1999]. Each mitral cell is connected to a single glomerulus and so its responses must be dominated by the activity in that glomerulus and, by inference, by the activity of the single odorant receptor present in the sensory neurons projecting to that glomerulus (Johnson et al., 1998). Mitral cells adjacent to each other project to the same or neighbouring glomerulus. Careful investigation of neighbouring mitral cells indicates that they have similar responses and that they tend to be activated by odorants of a similar molecular class, such as acids or ketones, or those containing a benzene ring (Mori et al., 1999). Mitral cells separated spatially are activated by odorants carrying different functional groups (Mori et al., 1999), an observation that would be predicted by the spatial differences in activity across the glomeruli (Johnson et al., 1998). Thus, the mitral cells appear to function as “molecular feature detectors”, a property brought about by the spatial segregation of inputs into the olfactory bulb by way of the singular expression of odorant receptors in individual sensory neurons and their targeting to distinct points in a spatial “map” on the surface of the olfactory bulb.
An understanding of this spatial map is now such that the neural map can be predicted from the structure of the molecule (Leon & Johnson, 2003). But is this information used by the animal? Evidence that it is comes from a study of the ability of rats to discriminate optical isomers (enantiomers), molecules that differ in structure as mirror images. Enantiomers that humans can identify as different (l- and d-carvone) activated different spatial maps of glomerular activity and were discriminated by rats (Leon & Johnson, 2003). Enantiomers that humans do not distinguish (l- and d-limonene, l- and d-terpinen-4-ol) activated similar spatial maps of glomerular activity and were not discriminated by rats in non-reinforced behavioural testing (Linster et al., 2002). It is notable that the variation of glomerular activity within the larger modules allows rats to discriminate otherwise similar enantiomers, if the learning is reinforced (Linster et al., 2001), and that glomerular activity is enhanced by such reinforcement (Coopersmith & Leon, 1984). This ability to learn to make use of small differences in glomerular activity may explain the remarkable ability of rats to learn to discriminate odorants even after large lesions of the olfactory bulb (Bisulco & Slotnick, 2003).
In summary, the functional anatomy of the primary olfactory pathway appears to impose a spatial component to the activity of cells within the olfactory bulb such that a “topographic map” of activity across the surface of the olfactory bulb is converted into a “chemotopic map” of molecular features extracted from the odorant stimulus. Our present level of understanding suggests that these molecular features are then reassembled into more complex representations of the stimuli, at least in the piriform cortex. In concept at least, this aspect of olfactory processing resembles that which occurs in the visual cortex whereby the visual image is initially decomposed into salient features in the responses of primary cortical neurons (features such as orientation, velocity and direction of movement, colour) before being reassembled into more complex representations of faces, hands or movement.
Other chemosensory systems in the nose
It is commonly known that “smelling” an odour is a function of the nose and that the “olfactory system” is responsible for this sensation, starting with the sensory organ, the olfactory mucosa, in the dorso-posterior part of the nasal cavity. Less well known is the contribution of the trigeminal nerve in odour sensation, especially in perceptions of odour “strength” and pungency.
The trigeminal nerve innervates the face, including the oral and nasal cavities, and the cornea and conjunctiva of the eye (Silver & Finger, 1991). In animal experiments, it is shown that the trigeminal nerve responds to many chemical compounds that are “odorous” to humans (Silver & Finger, 1991) and humans judged to have no functional olfactory system can detect a large range of odorants presented to the nose, presumably via the trigeminal nerve (Doty et al., 1978). Therefore, any consideration of olfactory anatomy and function must acknowledge the contribution of sensations via this “somatosensory” input.
Olfactory-guided behaviour, most notably food selection and eating, also involves the gustatory system as well as the olfactory and trigeminal systems. The gustatory system provides perceptions of sweet, sour, salty and bitter as well as umami, the taste of monosodium glutamate (Gilbertson et al., 2000). The trigeminal nerve in the mouth provides touch, temperature and “hot spicy” sensations, and the three sensory systems contribute to the perception of flavour, the “taste” of food. Commonly, persons reporting loss of “taste” of food actually have olfactory deficits (Doty et al., 1991). This is evident in human cerebral imaging experiments where the orbitofrontal cortex and insular cortex are activated by olfactory, gustatory and trigeminal stimuli.
© Cambridge University Press