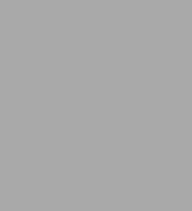
The Earth's Ionosphere: Plasma Physics and Electrodynamics
576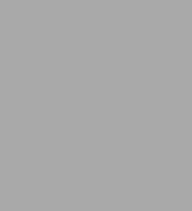
The Earth's Ionosphere: Plasma Physics and Electrodynamics
576eBook
Available on Compatible NOOK devices, the free NOOK App and in My Digital Library.
Related collections and offers
Overview
Product Details
ISBN-13: | 9780080916576 |
---|---|
Publisher: | Elsevier Science & Technology Books |
Publication date: | 06/12/2009 |
Series: | International Geophysics , #96 |
Sold by: | Barnes & Noble |
Format: | eBook |
Pages: | 576 |
File size: | 210 KB |
Read an Excerpt
The Earth's Ionosphere
Plasma Physics and ElectrodynamicsBy Michael C. Kelley
Academic Press
Copyright © 2009 Elsevier Inc.All right reserved.
ISBN: 978-0-08-091657-6
Chapter One
Introductory and Background Material
In this introductory chapter we present a qualitative treatment of several topics that we hope is sufficient to proceed with our study of ionospheric physics. The chapter begins with historical comments and a description of the limitations we have set for the text. In particular, we do not repeat or significantly update the material published by Rishbeth and Garriott (1969) earlier in this same International Geophysics Series. Rather, our emphasis is on electrodynamics and plasma physics, so we refer the interested reader to Rishbeth and Garriott and to Banks and Kockarts (1973) for more information about formation of the ionosphere, its ion chemistry, heat balance, and other aeronomic properties. Another and more recent resource for classical ionospheric science is the text by Schunk and Nagy (2000). Lyons and Williams (1984) have published a text on magnetospheric science.
1.1 Scope and Goals of the Text
1.1.1 Historical Perspective
The earth's ionosphere is a partially ionized gas that envelops the earth and in some sense forms the interface between the atmosphere and space. Since the gas is ionized, it cannot be fully described by the equations of neutral fluid dynamics. In fact, a major revolution in ionospheric physics has occurred in the past decades as the language and concepts of plasma physics have played an increasing role in the discipline. On the other hand, the number density of the neutral gas exceeds that of the ionospheric plasma, and certainly neutral particles cannot be ignored. A student of the ionosphere must thus be familiar with both classical fluid dynamics and plasma physics. Even a working knowledge of these two "pure" branches of physics, however, is not sufficient. Since the ionosphere lies at the interface between two very different and dynamic media, we must understand enough of both atmospheric dynamics and deep space plasma physics to understand how the ionosphere is formed and buffeted by sources from above and below. Added to these two is the requirement for a sufficient knowledge of ion and neutral chemistry and photochemistry to deal with production and loss processes.
Ionospheric physics as a discipline grew out of a desire to understand the origin and effects of the ionized upper atmosphere on radio wave propagation. The very discovery of the ionosphere came from radio wave observations and the recognition that only a reflecting layer composed of electrons and positive ions could explain the characteristics of the data. Most of the early work was aimed at explaining the various layers and their variability with local time, latitude, season, and so forth. The ionosonde, a remote sensing device that yields electron density profiles up to but not above the altitude of the highest concentration of charged particles, was the primary research tool. Such measurements revealed a bewildering variety of ionospheric behavior ranging from quiet, reproducible profiles to totally chaotic ones. Furthermore, a variety of periodic and aperiodic variations were observed, with time scales ranging from the order of the solar cycle (11 years) to just a few seconds. As time passed, the emphasis of ionospheric research shifted from questions dealing with formation and loss of plasma toward the dynamics and plasma physics of ionospheric phenomena.
Ionospheric research has greatly benefited from the space program with the associated development of instruments for balloons, rockets, and satellites (see Appendix A). The combination of remote ionospheric sensing and direct in situ measurements made from spacecraft has accelerated the pace of ionospheric research. Equally important has been the development of plasma physics as a theoretical framework around which to organize our understanding of ionospheric phenomena. Likewise, the continuing development of magnetospheric and atmospheric science has very much influenced our understanding of the ionosphere.
In parallel with these developments, the incoherent scatter radar technique was devised (see Appendix A), and several large facilities were built to implement it. The primary advantage of this method was the ability to make quantitative measurements of numerous ionospheric parameters as a function of altitude at heights that were inaccessible to ground-based ionosondes. Since interpretation of the scattered signal requires detailed understanding of the interaction between the electrodynamic waves and thermal fluctuations in a plasma, a working knowledge of plasma physics became necessary for understanding the diagnostics as well as the science.
Ionospheric science has thus evolved toward the point of view that is encompassed by the term space plasma physics. The central theme of this book is the treatment of ionospheric physics within this context, but many other important issues are not discussed, including the topside ionosphere, seasonal behavior, heat balance, and global morphology.
1.1.2 Organization and Limitations
As indicated in the previous section, our goal is to treat the electrodynamics and plasma physics of the ionosphere in some detail. We do not, therefore, have the space to start from the first principles of ionospheric science, particularly with regard to photoproduction, ion chemistry, recombination, and related topics. In this first chapter, we attempt a broad general introduction to this area, which we hope will provide enough background to make the remainder of the text meaningful.
It is also necessary to introduce elements of neutral atmospheric and magnetospheric physics, since the ionosphere is very much affected by processes that originate in these two regions. The ionosphere coexists with the upper portion of the neutral atmosphere and receives considerable energy and momentum from the lower atmosphere as well as from the magnetosphere. The energy and momentum fluxes are carried by particles, electromagnetic fields, and atmospheric waves. We devote some time in this first chapter to a qualitative description of the atmosphere and magnetosphere. Although useful as a starting point, the descriptive approach is not sufficient in all aspects, and we will treat some particularly important processes, such as gravity waves, in detail as they arise in the text.
Some knowledge about experimental techniques is necessary for a student of ionospheric dynamics, if only to understand the sources of the data. Accordingly, Appendix A is included, describing some of the most important measurement methods. The choice of instruments so dealt with is not exhaustive and certainly reflects the bias and expertise of the author.
Finally, we need to limit the scope of the text. We have somewhat regretfully chosen not to include a detailed discussion of the acceleration of auroral particle beams. Part of the rationale for this decision concerns the height range where the electron acceleration usually takes place, some 2000 km or more above the earth's surface, which is the limiting height range of the ionosphere as we define it here. Thus, even though particle acceleration can and does occur much lower than the 2000 km height, we consider this topic to be outside the domain of this book, if not always outside the height range under detailed consideration.
In Chapter 2 some basic equations and concepts are developed for use throughout the text. Some of these concepts would be quite useful as background for reading the rest of this first chapter, particularly the magnetospheric section. We thus recommend that a reader unfamiliar with the field read Chapter 1 twice, once for background and once again after reading Chapter 2. Chapters 3 and 4 deal with the electrodynamics and plasma physics of the equatorial ionosphere. This region is singled out, since the earth's horizontal magnetic field in that area leads to a number of unique phenomena. An interesting analogy exists with atmospheric and ocean dynamics. Since the Coriolis force vanishes at the equator, there are some unique phenomena common to both meteorology and oceanography that make equatorial dynamics very unusual and interesting. Chapters 5 and 6 deal with the tropical and midlatitude ionosphere, where the earth's magnetic field has a sizable inclination but is not vertical and usually does not link the ionosphere with the hot, tenuous, flowing plasmas of the magnetosphere or solar wind. Here, a toroidal region of relatively cold, dense plasma, called the plasmasphere or protonosphere, exchanges plasma with the ionosphere. In the remainder of the text after Chapter 6, we study the high-latitude region. As previously mentioned, several experimental techniques are described in Appendix A. Appendix B provides formulas and tables that will help the reader describe ionospheric phenomena. Appendix B also defines various magnetic activity indices used in the text.
1.2 Structure of the Neutral Atmosphere and the Main Ionosphere
Owing to the pervasive influence of gravity, the atmosphere and ionosphere are to first order horizontally stratified. Atmospheric structure can be neatly organized by a representative temperature profile, while the ionosphere is more sensibly organized by the number density of the plasma. Typical midlatitude profiles of temperature and plasma density are given in Fig. 1.1. The atmospheric temperature initially decreases with altitude from the surface temperature, with a "lapse rate" of about 7 K/km in the troposphere. At about 10 km altitude this temperature trend reverses (at the tropopause) and the stratosphere begins. This increase is due primarily to the absorption, by ozone, of part of the ultraviolet portion of the solar radiation. This effect maximizes at 50 km, where the temperature trend again reverses at the stratopause. Radiative cooling creates a very sharp temperature decrease to a minimum in the range 130–190 K at about 90 km. For heights above the altitude of the temperature minimum (the mesopause), the temperature increases dramatically due to absorption of even higher energy solar photons to values that are quite variable but are often well above 1000 K. Not surprisingly, this region is termed the thermosphere. The atmosphere is relatively uniform in composition below about 100 km due to a variety of turbulent mixing phenomena. Above the "turbopause" the constituents begin to separate according to their various masses.
The temperature increase in the thermosphere is explained by absorption of UV and EUV radiation from the sun. The EUV radiation is also responsible for the production of plasma in the sunlit hemisphere, since these solar photons have sufficient energy to ionize the neutral atmosphere. Equal numbers of positive ions and electrons are produced in this ionization process. One requirement for a gas to be termed a plasma is that it very nearly satisfies the requirement of charge neutrality, which in turn implies that the number density of ions, ni, must be nearly equal to the number density of electrons, ne. Experimenters who try to measure ni or ne usually label their results with the corresponding title, ion, or electron density. In this text, we shall usually refer to the plasma density n, where n [congruent to] ni [congruent to] ne is tacitly assumed to hold. Of course, if ni exactly equaled ne everywhere, there would be no electrostatic fields at all, which is not the case.
A note on units is in order here. Rationalized mks units will be used except in cases where tradition is too firmly entrenched. For example, measuring number density per cubic centimeter is so common that we shall often use it rather than per cubic meter. Likewise, the mho and the mho per meter will be used for conductivity. Some further discussion of units and of various parameters of interest to ionospheric physics is included in Appendix B.
(Continues...)
Excerpted from The Earth's Ionosphere by Michael C. Kelley Copyright © 2009 by Elsevier Inc. . Excerpted by permission of Academic Press. All rights reserved. No part of this excerpt may be reproduced or reprinted without permission in writing from the publisher.
Excerpts are provided by Dial-A-Book Inc. solely for the personal use of visitors to this web site.
Table of Contents
PrefaceChapter 1 Introductory and Background Material 1.1 Scope and Goals of the Text 1.1.1 Historical Perspective 1.1.2 Organization and Limitations 1.2 Structure of the Neutral Atmosphere and the Main Ionosphere 1.3 D-Region Fundamentals 1.4 The Earth's Magnetic Field and Magnetosphere 1.5 Problem SetReferences Chapter 2 Fundamentals of Ionospheric and Magnetospheric Plasma Dynamics 2.1 The Basic Fluid Equations 2.1.1 Conservation of Mass 2.1.2 Equation of State 2.1.3 Momentum Equation for the Neutral Fluid 2.1.4 Momentum Equations for the Plasma 2.1.5 The Complete Equation Sets 2.2 Steady-State Ionospheric Plasma Motions Due to Applied Forces 2.3 Generation of Electric Fields 2.4 Electric Field Mapping 2.5 Elements of Magnetospheric Physics 2.5.1 The Guiding Center Equations and the Adiabatic Invariants 2.5.2 Magnetohydrodynamics 2.6 Are Ionospheric Electric Fields Real? 2.7 Coordinate Systems 2.8 Problem SetReferences Chapter 3 Dynamics and Electrodynamics of the Equatorial Zone 3.1 Motions of the Equatorial F Region: The Data Base 3.2 The Equatorial F-Region Dynamo 3.3 E-Region Dynamo Theory and the Daytime Equatorial Electrojet 3.4 Further Complexities of Equatorial Electrodynamics 3.4.1 The Prereversal Enhancement 3.4.2 High-Latitude Effects on the Equatorial Electric Field 3.5 Feedback Between the Electrodynamics and Thermospheric Winds 3.6 Mesospheric and Lower Thermospheric Dynamics 3.6.1 Atmospheric Winds in the Mesosphere and Lower Thermosphere 3.6.2 A Primer on Turbulence and the Turbopause 3.7 Problem SetReferences Chapter 4 Equatorial Plasma Instabilities and Mesospheric Turbulence 4.1 F-Region Plasma Instabilities: Observations 4.2 Development and Initiation of Convective Ionospheric Storms (a.k.a. Equatorial Spread F) 4.2.1 Linear Theory of the Rayleigh-Taylor Instability 4.2.2 The Generalized Rayleigh-Taylor Process: Electric Fields, Neutral Winds, andHorizontal Gradients 4.2.3 The Seeding of Convective Ionospheric Storms by Gravity Waves 4.2.4 Role of Velocity Shear in Convective Ionospheric Storms 4.2.5 Summary of Linear Theory Results 4.3 Nonlinear Theories of Convective Ionospheric Storms 4.3.1 Two-Dimensional Computer Simulations 4.3.2 Simulations Including Seeding and Shear 4.3.3 Summary of Nonlinear Theory Results 4.4 Linkage of Large and Small Scales in CEIS 4.4.1 Evidence for a Diffusive Subrange 4.4.2 The Diffusive Subrange 4.4.3 Toward a Unified Theory for the Convective Ionospheric Storm Spectrum 4.5 Convective Ionospheric Storms Summary 4.6 E-Region Plasma Instabilities: The Observational Data Base 4.7 Linear Theories of Electrojet Instabilities 4.8 Nonlinear Theories of Electrojet Instabilities 4.8.1 Two-Step Theories for Secondary Waves 4.8.2 On the Observations that the Phase Velocity of Type I Equatorial Waves isIndependent of Angle 4.8.3 Nonlinear Gradient Drift Theories 4.8.4 Nonlinear Studies of Farley-Buneman (FB) Waves 4.9 D-Region Turbulence 4.10 Future Directions 4.11 Problem SetReferences Chapter 5 Hydro- and Electro-dynamics of The Mid-Latitude Ionosphere 5.1 Introduction to the Tropical and Mid-Latitude Ionospheres 5.1.1 Background Material 5.1.2 On the Height of the Daytime F2 Layer 5.1.3 Equations Including Vertical Flux Without Winds or Electric Fields 5.1.4 F-Layer Solutions with Production, Diffusion, and Flux 5.1.5 More General Nighttime Solutions 5.1.6 The Appleton Anomaly: An Equatorial Electric Field Effect 5.1.7 The Corotation Electric Field and Formation of the Plasmasphere 5.2 Electric Fields in the Tropical and Mid-Latitude Zone 5.2.1 Electric Field Measurements 5.2.2 Neutral Wind Effects 5.2.3 Combined Effects of Electric Fields and Neutral Winds 5.2.4 Complexities of the Real Nighttime Tropical Ionosphere 5.2.5 The Transition Zone between Mid and High Latitudes 5.3 Mid-Latitude Lower Thermosphere Dynamics 5.3.1 Tidal Effects 5.3.2 Wind Profiles 5.4 Problem SetReferences Chapter 6 Waves and Instabilities at Mid-Latitudes 6.What People are Saying About This
The classic text newly available again in a fully up-to-date second edition