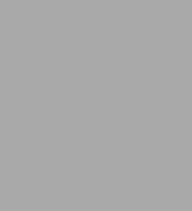
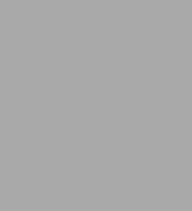
eBook
Available on Compatible NOOK devices, the free NOOK App and in My Digital Library.
Related collections and offers
Overview
Product Details
ISBN-13: | 9780486150093 |
---|---|
Publisher: | Dover Publications |
Publication date: | 11/21/2012 |
Sold by: | Barnes & Noble |
Format: | eBook |
Pages: | 240 |
File size: | 19 MB |
Note: | This product may take a few minutes to download. |
Read an Excerpt
The Nature of Visual Illusion
By Mark Fineman
Dover Publications, Inc.
Copyright © 1981 Oxford University Press, Inc.All rights reserved.
ISBN: 978-0-486-15009-3
CHAPTER 1
A Light and Color Primer
Almost everyone has seen color samples in a paint store, those small squares of color arranged in neat progessions on cards. A paint manufacturer provides his customers with several hundred samples at most, but imagine for a moment that someone set out to create every possible color that the human eye could distinguish. How many colors would there be? Thousands? Tens of thousands? Actually, the number of discriminably different colors has been estimated to be about 7.5 million!
How is that possible? Are there over seven million different kinds of receptors in the eye? Is light itself made up of millions of colors? Color vision is a good topic with which to start an examination of visual perception because it illustrates many of the complexities peculiar to the larger subject of vision. Before we try to understand the workings of color vision, however, it would be a good idea to consider a few fundamentals of vision.
VISUAL PERCEPTION
In the simplest of definitions, visual perception can be reduced to three events: 1. the presence of light, 2. an image being formed on the retina, and 3. an impulse transmitted to the brain.
1. Vision requires a stimulus. This stimulus is normally in a form of energy called light. Although sometimes a person claims to see in the absence of light, as in a dream or hallucination, these are special instances that do not pertain to the immediate discussion.
2. The light stimulus enters the eye, where it is refracted (bent) in such a way that an image is formed on the interior of the eye, a light sensitive surface known as the retina. The image-forming parts of the eye are the cornea, a clear bulge at the front of the eye, and the lens, located within the eye a short distance behind the cornea. You can see someone else's cornea by asking that person to stare straight ahead while you observe his eye from the side.
The iris and pupil mechanism, interposed between cornea and lens, regulates the overall level of light that enters the eye. In bright light the pupil constricts, and in dim light it dilates. The pupil itself is an aperture whose diameter is controlled by the surrounding colored iris. The lens and the retina are on the interior of the eye and cannot be seen just by looking at the exterior of another person's eye.
The retina is composed of millions of specialized receptor cells, as well as other types of cells that support the transmission from the retina to the brain. The receptors respond to the light striking them (the image) by triggering a chain of chemical reactions. It is this series of chemical changes that is transmitted from cell to cell, from retina to brain.
3. Thus the third event is the transmission of this chemical impulse to the brain, causing further chemical changes in the billions of cells that constitute the brain. These alterations in brain activity are unquestionably at the core of our responses to light, responses that can be conveniently categorized as "seeing."
To summarize then, any time we talk about visual perception, three events must occur: a stimulus, an image, and the transmission of the impulse to the brain. Because of this, an analysis of any visual phenomenon—including color vision—can be made at one or more of these levels. In this chapter I would like to pay particular attention to some basic features of color vision, while at the same time noting some general features of visual perception.
LIGHT AND COLOR
The visual stimulus, light, is one manifestation of electromagnetic energy, which also encompasses such familiar phenomena as X rays, and radio and television transmissions.
Electromagnetic energy can be pictured as having the regular undulations of a wave, one whose distance from peak to peak may vary. For this reason it is common practice to describe an electromagnetic phenomenon in terms of its wavelength. If electromagnetic energy were arrayed according to wavelength, from shortest to longest, so as to form a spectrum, X rays would occur at the short end, broadcast bands would be at the long end, and the waves to which vision responds (visible light) would occupy an intermediate position. In fact, the wavelengths of visible light vary from about 300 to 700 nanometers. Since a single nanometer is a mere billionth of a meter long, it can be quickly appreciated that waves of visible light are still quite short.
In addition, electromagnetic energy (including visible light) has particle properties. When applied to light, a particle or quantum of energy is called a photon. This photon is often conjectured to be like a tiny packet of energy, one that oscillates in waveform as it travels.
No one is entirely certain why it is that we respond exclusively to the relatively tiny portion of the electromagnetic spectrum occupied by visible light. Some theorists have speculated that since these wavelengths are abundant in the sun's radiations, it is reasonable to suppose that we would have evolved to be sensitive to them. It should be noted that some species of animals actually respond to slightly longer or shorter wavelengths than those of visible light.
Light at different wavelengths within the visible part of the spectrum varies in color. Longer wavelengths look red and shorter wavelengths look blue. Surely everyone is familiar with Sir Isaac Newton's classic experiment: In an otherwise darkened room, a beam of sunlight was allowed to pass through a slit in a window-shade. The beam then passed through a glass prism and finally on to a screen. The prism had the effect of separating out the component wavelengths of the original beam of white light (light which contained all wavelengths in roughly equal proportions), creating a spectrum of visible wavelengths. Nature repeats substantially the same experiment whenever a rainbow appears.
Thus we can see a basis for color vision in the stimulus itself, and one might be inclined to assume that color vision is largely a matter of detecting various wavelengths of light. Even though this hypothesis is attractive, it still cannot account for the complexity of color vision. Remember that there are only about 400 wavelengths of visible light, yet we see millions of color tints and shadings. Even if we could discriminate every wavelength of visible light, we could account for the perception of no more than a few hundred colors at best.
INTENSITY AND BRIGHTNESS
The intensity of light is a measure of its energy. It is calculated by multiplying the frequency of light by a constant, named for the eminent German physicist Max Planck who discovered it, and which is therefore called Planck's constant. Now you might suppose that if the energy of light were increased, we would always report that the light appeared brighter. In fact, however, the brightness of an object is only partially related to the energy of the light given off by that object. The intricacy of the intensity-brightness relationship is implicit in the word brightness itself, since brightness refers to how people see or respond to the energy dimension rather than to the energy itself. You may find it convenient to think of brightness as a measure of perceived intensity. Many researchers consider brightness perception to be an integral part of color vision.
Why has it been necessary to construct this confusing concept of brightness? Why not stick with a straightforward measure of light energy? One reason is shown in the accompanying illustration, in which several gray squares are enclosed by larger squares. In every case the interior squares reflect equal levels of light to the viewer's eyes, a fact that could be easily verified by measuring them with a light meter, or by inspecting the interior squares without the surrounding squares. For example, cut out the white mask and place it over the illustration so that only the inner squares can be seen. When viewed against the uniform white background, the interior squares should appear equally bright. Even though the inner squares are of equal intensity, they nonetheless appear to be of unequal brightness values when the surrounding squares are in view. This contrast effect is attributable to the viewer's own visual system and will be discussed again in later chapters.
One of the reasons that color vision is so difficult to fathom is that both the nature of light and the nature of the observer's visual system must be considered, as these illustrations may have already suggested. Therefore, let's turn to some of the complications created by the interaction of stimulus and observer.
COLOR MIXING AND COLOR VISION
More than a century ago, Thomas Young, the English physicist and physician, suggested that lights of any three different colors may be combined to create all of the remaining colors of the visible spectrum. For example, if a beam of green light is projected on to a white screen, and a beam of red light is made to overlap the first beam on the screen, the overlapped region will appear yellowish. Thus three lights and their combinations are all that would be needed to create all of the colors of the visible spectrum. In the red and green example, the receptors of the retina are stimulated by wavelengths at two locations on the spectrum, and so this is an example of an additive color mixture. (Additive mixtures are typical of the human eye.)
Most people, however, are more familiar with a subtractive color mixture such as the type that occurs when paints or pigments are combined. Mixing blue paint with yellow paint yields green. It is called a subtractive mixture because the combination of paints acts to absorb light in certain portions of the spectrum while allowing light at the remaining wavelengths to be reflected to the eye.
The important consideration is that only three colors and their combinations are needed to create all of the spectral colors. Young further theorized that there need be only three types of color receptors in the eye, each responsive to a different portion of the spectrum. Their combined activity could then account for the remaining colors. This trichromatic theory of color reception has now been amply supported by the evidence of many laboratory studies, and there is no need to postulate the existence of scores of different cell types in the retina—let alone millions—in order to account for color vision at the level of the eye.
On a more practical level, our knowledge of color mixture and color vision has made color photography possible, as well as color television and color printing. Color film, for example, is a sandwich of three photosensitive layers, each of which reacts chemically to a different portion of the spectrum. The mechanism by which color film operates is therefore analogous to that of the color receptors in our eyes.
COLOR INTERACTIONS
Although the trichromatic theory helped to simplify our understanding of color receptors, it didn't answer all the questions. There are some colors that do not appear in the spectrum arrayed by Newton's prism. Where do colors like pink and brown fit in? Metallic colors (such as silver and bronze) and shades of gray also bear no obvious relationship to the spectrum.
Part of the answer lies in the fact that colored light may be combined with white light to varying degrees. Red light combined with white light will appear lighter, more "pinkish." The grays are a function of intensity of white light and can only be seen as surfaces, not in lights.
Colors can also interact in many ways. One way to see a color interaction is to repeat the earlier contrast demonstration substituting colored paper squares for the gray patches. Any kind of uniformly colored paper will do. Cut out several squares of the same color (a pale green works well) and see how the squares compare when placed against patches of other colors. You should be able to detect subtle differences in brightness, as before, but also slight differences in tint as well.
COLOR CONSTANCY
Understanding color vision is also complicated by a characteristic of observers called hue constancy or color constancy. So far we have assumed that the light ordinarily illuminating our environment is white light, containing roughly equal proportions of light from all of the visible wavelengths. In actuality white light is the exception rather than the rule. Sunlight only approaches the white standard around noontime. At other times of the day its spectral composition is more varied due to the filtering properties of the atmosphere; sunlight passes through varying thicknesses of atmosphere at different times of the day, and the color of sunlight may be affected by dust particles and pollutants suspended in the air. Toward sunrise and sunset light has relatively more energy at the red end of the spectrum and somewhat less at shorter wavelengths than is typical at high noon.
Most lightbulbs also emit a spectrum that is biased from true white light. Incandescent bulbs are reddish, while fluorescent tubes are deficient in long wavelengths and therefore emit a spectrum that is bluish when compared with white light.
We are not normally aware of these discrepancies from white light. Even though objects reflect varying percentages of the visible wavelengths under different conditions of illumination, color perception remains constant. Common objects look much the same whether seen by sunlight, lightbulb, or fluorescent tube. The visual system seems to disregard minor discrepancies in spectral composition so that colors appear much as they would under white light illumination. In this way we are able to maintain a stable world of color under changing conditions of illumination.
One way to demonstrate the constancy of color vision is to take color photographs of the same scene under different conditions of illumination. The best film to use is color slide film, such as Kodak's Kodachrome or Ektachrome, both of which are said to be "balanced" for sunlight at noon. Unlike the visual system of humans, color film cannot adapt to shifts in the coloration of the illuminating light, and its appearance will change as the color quality of the light changes. Try making the following comparison: Take a series of pictures of the same subject at regular intervals throughout the day. You will find that the presence of a few normally white objects in the scene will help to evaluate the results. You may be surprised to see dramatic color changes from picture to picture, changes you had not even been aware of while taking the pictures. Next, take some pictures indoors with the same film, using the light of electric fixtures as the sole illuminant. What would you predict about the appearance of color in pictures that had been illuminated by lightbulbs, as compared with fluorescent illumination?
The adaptability of color vision has its limits. While wide fluctuations in color quality are tolerated in vision, too narrow a band of wavelengths is unacceptable for normal perception of color. To prove this point, obtain a good quality red or green lightbulb and try to identify the colors in magazine illustrations using the bulb as the sole source of illumination. This is a little tricky since you will have had some acquaintance with the colors of many of the objects depicted in the magazine. If you remain perfectly objective, however, you will see that the magazine looks dramatically different under the uniform illumination. If you use a red bulb, the blue printed areas of the page will appear dark. Because much of the light emitted by the bulb is in the long end of the visible spectrum, the red dyes on the printed page will continue to reflect light to the eye, but the bulb emits few wavelengths at the blue end of the spectrum and so there is nothing for the blue dyes to reflect. Therefore areas that looked blue under normal illumination now appear almost black under red light illumination.
The peculiar appearance of colors under homogeneous color illumination is much the same as happens with certain kinds of street lighting, such as the newer sodium or mercury vapor lamps, which emit light only wtihin a narrow band of wavelengths.
(Continues...)
Excerpted from The Nature of Visual Illusion by Mark Fineman. Copyright © 1981 Oxford University Press, Inc.. Excerpted by permission of Dover Publications, Inc..
All rights reserved. No part of this excerpt may be reproduced or reprinted without permission in writing from the publisher.
Excerpts are provided by Dial-A-Book Inc. solely for the personal use of visitors to this web site.
Table of Contents
1. A Light and Color Printer2. The Minimum Case for Vision
3. Completion: Phantoms of the Visual System
4. Motion Receptors
5. The Illusion of Movement
6. The Wagon Wheel Effect
7. Motion Perception: Learned or Innate?
8. Kinetic Art
9. More Kinetic Depth
10. Leonardo's Window
11. The Size Problem
12. Two Eyes Are Better than One
13. Pulfrich's Amazing Pendulum
14. The Anamorphic Puzzle
15. The Probability of Form
16. The Restless Eye
17. "Bands, Grids, and Gratings"
18. Color Curiosities
19. Poggendorf's Illusion
Annotated Bibliography
Index