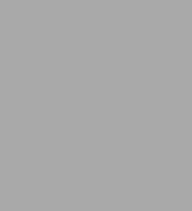
Learning with Animation: Research Implications for Design
402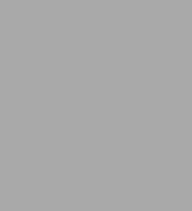
Learning with Animation: Research Implications for Design
402Hardcover
-
SHIP THIS ITEMIn stock. Ships in 1-2 days.PICK UP IN STORE
Your local store may have stock of this item.
Available within 2 business hours
Related collections and offers
Overview
Product Details
ISBN-13: | 9780521851893 |
---|---|
Publisher: | Cambridge University Press |
Publication date: | 11/19/2007 |
Pages: | 402 |
Product dimensions: | 5.98(w) x 9.02(h) x 1.06(d) |
About the Author
Richard Lowe is Professor of Learning Technologies at Curtin University in Perth, Australia. Following undergraduate studies in chemistry and education, he completed a Ph.D. in educational psychology at Murdoch University. As a result of his work in industry, education, and textbook publication, he developed an interest in factors influencing the effectiveness of explanatory graphics. From an early focus on the comprehension of static graphics, his research has extended in recent years to include investigations of the educational effectiveness of animated and interactive graphics. In addition to his research, he continues to work as a practicing instructional designer for industry and government organizations that rely on complex and dynamic graphic information displays for their operations. He is currently Associate Editor for the international journal Educational Research Review.
Read an Excerpt
Cambridge University Press
978-0-521-85189-3 - Learning with Animation - Research Implications for Design - Edited by Richard Lowe and Wolfgang Schnotz
Excerpt
SECTION ONE
INFORMATION SEARCH AND PROCESSING
1
Effects of Knowledge and Spatial Ability on Learning from Animation
Mary Hegarty and Sarah Kriz
INTRODUCTION
Static diagrams have been used to present scientific and technical information since the invention of the printed book (Ferguson, 1992). However, animations are a relatively recent graphic invention. With the growth of computer technology in everyday life, educational settings, and in the workplace, the ability to communicate information through animation is increasing. However, to use this technology effectively in communication, education, and training, we need to understand how and under what circumstances people learn from animations.
Initial results on the effectiveness of animations have been disappointing, suggesting that they are no more effective than static diagrams. For example, in a review of dozens of studies that compared learning from animations and static media, Tversky Morrison, andBétrancourt (2002) found no evidence for an advantage of animations over static diagrams when the information presented in the two media was controlled. A common response to this result is to assume that the animations used in early research were poorly designed, so that the solution is to improve the design of animations. Thus, several researchers have suggested principles for the design animations, including adding interactive control, adding devices to draw the user’s attention to the most important information in the animation, and adding explanatory text (Faraday & Sutcliffe, 1997a; 1997b; Mayer, 2001; Tversky et al., 2002). When based on theory and research in cognitive psychology, these are referred to as cognitive design principles.
This current emphasis on ways of improving animations implicitly assumes a bottom-up model of animation comprehension. According to this account, comprehension is primarily a process of encoding the information in the external display, so that improving that display necessarily improves understanding. In contrast, less attention has been given to how learning from animations is affected by learners’ abilities, skills, prior knowledge, and misconceptions, that is, top-down influences on comprehension. In this chapter, we take an individual differences approach to examining learning from animations. Rather than assuming that there is a “best” type of information display that is equally effective for all learners, we start with the premise that individuals will bring different abilities, skills, and knowledge to the comprehension process, so that different types of information displays (animated vs. static; different types of animations) may be effective for different learners. We review a series of studies in which we examined the effects of spatial ability and prior domain knowledge on learning from animations, and derive recommendations about how to use animation effectively for different types of individuals.
Our research focuses on the use of animations to communicate how machines work. This is a good place to start looking at the effects of animation, because mechanics is essentially about movement. Understanding a machine involves developing an internal representation of the shapes and composition of the parts of the system, and how they are connected and arranged in space, that is, the configuration of the machine. But more critically, it includes knowledge of how the components move and affect each other’s motions, that is, the behavior of the machine. Central to this is knowledge of the causal chain or chains of events in the machine’s behavior. It includes both kinematic understanding of how the different machine components move in space and affect each other’s movements, and dynamic understanding of the forces that bring about these movements. Finally, it involves understanding the function of the machine, that is, what it is designed to do, and how the configuration and behavior achieve this function This knowledge comprises an individual’s internal representation or mental model of a machine (Chi, de Leeuw, Chiu, & LaVancher, 1994; Hegarty & Just, 1993; Narayanan & Hegarty, 1998).
Any display (e.g., diagram, animation, or text) that depicts or describes a machine is an external representation of the machine, that is, a representation that exists in the world rather than in the mind. Different types of external representations vary in how much information they represent about the machine, in how explicitly that information is represented, and in the type of mapping between the external representation and its referent (cf. Palmer, 1978). For example, a static diagram such as the one in Figure 1.1 can explicitly represent the configuration of the machine. This diagram is isomorphic to the machine that it represents, in the sense that the shapes of the parts correspond to the shapes of components of the machine, and the spatial relations between the parts correspond to the spatial relations between machine components. In contrast to a static diagram, an animation can explicitly represent both the configuration and kinematics of the machine. That is, in an animation, the movements of parts of the animated diagram are isomorphic to the movement of parts of the actual machine. Because force is not a visible entity, neither static nor animated diagrams can represent dynamics in a way that is isomorphic to reality. However, both static and animated diagrams can be accompanied by linguistic descriptions, which are more abstract in that they do not resemble the entities that they represent, and their comprehension depends on knowledge of conventions, such as the meaning of words. Linguistic descriptions have more expressive power than diagrams (Oestermeier & Hesse, 2000), so they may be better able to describe abstract ideas, such as the non-visible forces underlying the kinematics of a machine. Non-visible entities, like force, can also be represented by arrows, which are also conventional symbols.
Narayanan and Hegarty (1998) proposed a model of the process of understanding a machine from static diagrams and text. According to this model, people construct a mental model of a machine by first decomposing it into simpler components, retrieving relevant background knowledge about these components, and mentally encoding the relations (spatial and semantic) between components to construct a static mental model of the configuration of the machine parts. They then transform this static mental model to a kinematic/dynamic model, which represents how the components move and constrain each other’s motion when the machine is in operation. This process, which we term mental animation, is accomplished by determining the causal chain of events in the system and inferring the motion of machine components sequentially, in order of the causal chain, using either spatial visualization processes or rule-based reasoning (Hegarty, 1992; Narayanan, Suwa, & Motoda, 1994).
This model can be seen as an extension of constructivist theories of text processing (e.g., Chi et al., 1994; Graesser, Singer, & Trabasso, 1994; Kintsch, 1988) and is related to a more general model of multimedia comprehension proposed by Schnotz (2002). These theories view comprehension as an interplay between top-down and bottom-up process in which the learner encodes the new information in a text and integrates it with his or her prior knowledge of the domain. In addition to text comprehension skills, our model proposes that comprehension is dependent on spatial abilities and skills for integrating information in text and graphics, and encoding and inferring information from graphic displays (Hegarty & Sims, 1994). The model therefore predicts that what one understands from text and graphics is dependent on one’s prior domain knowledge and spatial abilities, in addition to the information presented in the display.
In this chapter, we examine learning from animations in the context of this comprehension model. We first review theory and research concerning the effects of spatial ability and domain knowledge on perception, comprehension, and learning from diagrams and animated displays. We then review some of our recent research on comprehension of animations alone, focusing on whether and how spatial ability and domain knowledge affect their comprehension. Next, we consider our recent research on comprehension of multimedia displays that include both diagrams (static and animated) and text, again focusing on the effects of spatial ability and knowledge. Finally, we make recommendations about how to best use animations in instruction about machines for individuals with different knowledge and ability levels.
REVIEW OF PRIOR LITERATURE
Effects of Spatial ability
In this section, we review theory and prior research regarding the effects of spatial ability on comprehension of animations. Although there are several subcomponents of spatial ability (see Hegarty & Waller, 2005; Lohman, 1988; McGee, 1979) in this review, we will focus on spatial visualization ability. Tests of this ability involve imagining a series of spatial transformations of an object (such as the folding and unfolding of pieces of paper) or the mental transformations of complex three-dimensional figures (for example mental rotation of 3D objects). Cognitive analysis of performance on tests of spatial ability has suggested that individual differences in performance on these tests reflect differences in speed of processing spatial information (Salthouse, 1996), spatial working memory capacity (Shah & Miyake, 1996; Miyake et al., 2001 ), and strategies for processing spatial information (Lohman, 1988; Just & Carpenter, 1985 ). More generally, spatial visualization ability has been characterized as the ability to construct and maintain high-quality internal spatial representations (spatial images) and to accurately transform these representations (Hegarty & Waller, 2005 ).
Animations and static diagrams can be thought of as external visualizations (external visual-spatial representations) and spatial ability can be thought of as internal visualization ability. Thus, effects of spatial ability on learning from animations can be framed in terms of in terms of the interplay between perception of external visualizations and internal visualization processes (Hegarty, 2004a). This is most evident in considering the comprehension processes involved in understanding kinematic and dynamic processes from animated versus static diagrams. Because an animation of a machine shows how it moves, the process of constructing an internal model from an animation depends on perception of motion. In contrast, constructing a mental model of a machine from static diagrams depends on inference of motion, which at least sometimes is a spatial visualization process (Hegarty, 2004b).
How might we expect spatial ability to influence learning from animations and other external visualizations? In the psychometric literature, spatial ability has been found to be highly correlated with mechanical ability (Bennett, 1969), which is not surprising given that an accurate mental mode of a mechanical systems must include a representation of spatial properties such as shape of components, configuration, and movement. Thus, even without instruction, people with high spatial ability are better able to understand mechanical processes. In any study that involves comprehension of mechanical systems, we might therefore expect a main effect of spatial ability on comprehension outcomes, and many studies of learning from text, diagrams, and animations have shown this effect (e.g., Hegarty & Just, 1993; Mayer, 2001).
A more interesting question concerns possible aptitude-treatment interactions (cf. Chronbach & Snow, 1977) between spatial abilities and the format of instruction. One possibility is that an external visualization such as an animation can compensate for lack of internal spatial visualization ability. More specifically, viewing an animation on a computer may compensate for lack of mental animation ability. If this were true, an animation could act as a cognitive “prosthetic” for those with low spatial abilities and we predict an aptitude treatment interaction such that low-spatial individuals would learn relatively more from animations than high-spatial individuals (who presumably don’t need to view external animations because they can mentally animate).
A second possibility is that spatial visualization ability may be a requirement for accurate perception and comprehension of external visualizations such as animations. For example, because spatial visualization partially reflects speed of processing (Salthouse, 1996), it might affect speed of encoding spatial information from an animation, such that if the animation is played a relatively fast speed, only high-spatial individuals will be able to keep up with the pace. Furthermore spatial visualization ability is related to greater spatial working memory capacity (Shah & Miyake, 1995; Miyake et al., 2001) which may be necessary to internally maintain spatial information presented at different stages of an animation so that it can be integrated and related in memory. Thus, perception and comprehension of an animation may depend on the same spatial abilities as mental animation. If this is the case, we expect an interaction such that animations are more effective for high-spatial individuals than low-spatial individuals.
A third possibility, is that external animations augment internal visualizations, that is, provide information or insights that are additional to those that can be provided by internal visualizations. For example, an external visualization might show a more complex process than can be internally visualized within the limited capacity of visual-spatial working memory. In this case, we might expect animations to be effective in communicating about dynamic processes for both high- and low-spatial individuals.
Finally, it is possible that external animations may impede comprehension for both high- and low-spatial individuals. Previous research on mental animation has suggested that people conceptualize the movement of a mechanical system as a causal series of events, such that the motion of each component in a mechanical system affects the motion of the next component in the causal chain (Hegarty, 1992; Hegarty & Sims, 1994). In contrast, a realistic animation shows all components moving continuously. Therefore, comprehension may be impaired by a mismatch between internal and external representations (Tversky et al., 2002).
Previous research on mechanical comprehension does not uniformly support any one of these possibilities. On the one hand, research in naïve physics suggests that under some circumstances, people’s judgments of mechanical events are more accurate if they view an animated rather than a static diagram. For example, McCloskey, Caramazza, and Green (1980) found that many people incorrectly predict that a ball emerging from a curved tube will continue to move in a curved trajectory (in fact it moves in a straight path). This can be interpreted as a failure to correctly mentally animate the movement of the ball. Kaiser, Proffitt, and Anderson (1985) later showed that if people with this misconception are shown different animations of a ball emerging from a curved tube, some showing an incorrect curved path and one showing the correct straight path, they are able to choose the animation that shows the correct path. Thus, people have superior performance when they see an animation than when they see a static view and imagine the motion. Kaiser et al. (1985) did not measure spatial ability in this research, so it is unclear whether these animations augmented cognition for all individuals, or were more effective for people with low or high spatial ability.
On the other hand, some studies suggest that spatial ability may enhance one’s ability to perceive or comprehend an animation. For example, Isaak and Just (1995) studied ability to judge the trajectory of a point on a rolling ball, which involves both translation and rotation. In this situation, people are subject to an illusion called the curtate cycloid illusion, which can be explained by a model in which they temporarily fail to process the translation component of motion at a critical point in the rolling motion. Isaak and Just found that people with high spatial visualization ability were less subject to the illusion than people with low spatial visualization ability. They proposed that spatial working memory, necessary for generating internal visualizations, was also necessary to simultaneously process the rotation and translation components of motion in perception of the external display, to accurately understand how the ball rolled.
Learning from external visualizations may also depend on spatial abilities. Mayer and Sims (1994) examined the role of spatial ability in learning from animations that explain how mechanical and biological systems work. They considered two alternative hypotheses. The first hypothesis was that viewing an animation would compensate for low spatial ability. The second hypothesis was that spatial ability would be a necessary prerequisite for learning from an animation. The results were consistent with the second hypothesis – high-spatial individuals learned more from the animations than low-spatial individuals. A later experiment indicated that this was only true if the animation was well designed, such that the commentary and graphics were presented simultaneously. With poorly designed animations in which these were presented sequentially, high spatials learned no more than low-spatial participants (Mayer, 2001).
Finally, in the field of medical education, Garg, Norman, Spero, and Maheshwari (1999) found that viewing three-dimensional rotations of carpal bone configurations impaired spatial understanding of the anatomy for low-spatial students whereas it improved understanding for high spatials. In a later experiment, they found that giving the user control of the animation eliminated this effect, so that both high- and low-spatial students learned from the animation. It appears, therefore, that spatial visualization ability may be a necessary prerequisite for learning from some external animations, but that the size of this effect depends on the design of the animation.
Effects of Prior Knowledge
In this section, we review theory and prior research regarding the effects of knowledge on comprehension of animations. Chi (2000) has identified four types of prior knowledge that learners may possess: domain-specific knowledge, domain-relevant knowledge, misconceptions, and domain-general world knowledge. With respect to domain-general world knowledge, Vosniadou (2002) has proposed that over the course of a lifetime, one’s perceptual experiences become organized into an explanatory framework, often referred to as naïve physics understanding, which often includes misconceptions, for example the belief that physical objects are solid and stable. Everyday interactions with machines may also affect our naïve physics understanding. In discussing effects of prior knowledge on learning, therefore, it is important to distinguish between domain-specific prior knowledge and domain-general world knowledge, and not simply “high” or “low” levels of prior knowledge. For example, knowledge acquired from physics classes may have different effects on learning than knowledge acquired from practical experience interacting with machines.
How might knowledge affect learning from animations? On the one hand, we might expect animations to be relatively more effective for low-knowledge individuals. Inference of motion from static diagrams can depend on knowledge as well as spatial visualization abilities (Naryanan & Hegarty, 1998; Hegarty 2004b; Schwartz & Black, 1996). Animations may be relatively more effective for those with low prior knowledge, because animations show the motion in a mechanical system explicitly, and do not rely on the learner’s ability to infer motion from static diagrams. Again, we can conceptualize this as a tradeoff between perception of an external representation and internal inference processes.
However, there are also several reasons to expect that comprehension of animations may be enhanced by prior domain knowledge. Constructivist theories of comprehension (e.g., Chi et al., 1994; Graesser et al., 1994; Kintsch, 1988; Schnotz, 2002) assume that learning involves the integration of new information into existing knowledge structures. The result of this integration process depends not only on how the new information is presented, but also on the quantity, specificity, and accuracy of the existing knowledge. In studies of verbal comprehension, students with low domain knowledge have trouble interpreting events in terms of the larger goal structure of the discourse, so that they remember fewer macrostructural propositions or main ideas (Chiesi, Spilich, & Voss, 1979; Hambrick & Engle, 2002). It seems reasonable to assume that prior knowledge may have similar effects to learning from anima-tions.
Prior domain knowledge may also help a learner recognize what he or she does not understand when acquiring new information. Miyake (1986) has proposed that developing a mental model of a mechanical system is often an iterative process of understanding, in which learners move back and forth between states of understanding and misunderstanding as they gain deeper understanding of how the system works. In this process, revising a mental model can only occur when a conflict between the external representation and the internal representation is perceived (Chi, 2000). A learner with a high level of prior domain knowledge should be better able to assess these “gaps” in their internal models or conflicts between internal and external representations. In contrast, low domain knowledge learners may be overconfident in judging their understanding of mechanical phenomena, as proposed by Rozenblit and Keil (2002). Because of this overconfidence, “novice scientists” may not perceive any gaps or inconsistencies between their internal models and external animations.
© Cambridge University Press