This book is a timely addition to the literature, as the first in the field, dedicated to detecting this molecule in vivo. It will be welcomed by the community particularly medicinal and analytical chemists, developers of sensors and probes and analytical equipment manufacturers.
This book is a timely addition to the literature, as the first in the field, dedicated to detecting this molecule in vivo. It will be welcomed by the community particularly medicinal and analytical chemists, developers of sensors and probes and analytical equipment manufacturers.
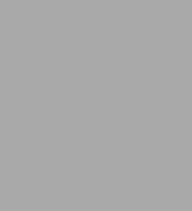
Peroxynitrite Detection in Biological Media: Challenges and Advances
284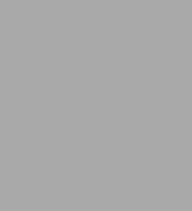
Peroxynitrite Detection in Biological Media: Challenges and Advances
284Hardcover
-
SHIP THIS ITEMNot Eligible for Free ShippingPICK UP IN STORECheck Availability at Nearby Stores
Available within 2 business hours
Related collections and offers
Overview
This book is a timely addition to the literature, as the first in the field, dedicated to detecting this molecule in vivo. It will be welcomed by the community particularly medicinal and analytical chemists, developers of sensors and probes and analytical equipment manufacturers.
Product Details
ISBN-13: | 9781782620853 |
---|---|
Publisher: | RSC |
Publication date: | 10/21/2015 |
Series: | Detection Science , #7 |
Pages: | 284 |
Product dimensions: | 6.15(w) x 9.20(h) x (d) |
About the Author
Sabine Szunerits is since 2009 Professor in Chemistry at the UniversityLille 1 and attached to the Interdisciplinary Research Institute (IRI). She holds a PhD in organic electrochemistry from the Queen Mary and Westfield College, London UK and a MA in Social Science from the Open University of London. She has become a member of the “Institut Universityaire de France” (IUF) in 2010. Her current research interests are in the area of material science with emphasis on the development of novel analytical platforms for the study of affinity binding events and in the modification of nanostructures for biomedical applications. She is co-author of more than 160 research publications, wrote several book chapters and has 6 patents.
Read an Excerpt
Peroxynitrite Detection in Biological Media
Challenges and Advances
By Serban F. Peteu, Sabine Szunerits, Mekki Bayachou
The Royal Society of Chemistry
Copyright © 2016 The Royal Society of ChemistryAll rights reserved.
ISBN: 978-1-78262-235-2
CHAPTER 1
Peroxynitrite: The Basics
WILLEM H. KOPPENOL
1.1 History
1.1.1 Before 1990
In this section, I will focus on the early discovery of peroxynitrite, its ability to nitrate aromatic compounds, its sensitivity to carbon dioxide and early determination with permanganate.
More than a 100 years ago, Baeyer and Villiger proposed that a "Nitrosopersäure" (ROONO) was formed as an intermediate in reactions of nitrite, ethyl nitrite, and amyl nitrite (R1ONO) with hydrogen peroxide and ethyl hydroperoxide (R2OOH). They came to the conclusion that an adduct between R1ONO and R2OOH was formed that yielded R1OH and R2ONO2. Had a direct oxidation of the nitrite taken place, R1ONO2 and R2OH would have been the products. In the case of the reaction between nitrous acid and hydrogen peroxide, nitrate (NO3-) is ultimately formed and the accompanying formula shows an adduct between peroxynitrous acid and water. Although they did not provide direct evidence for the structure of peroxynitrous acid, we may credit them with the discovery of this reactive species. In 1907, Raschig prepared a solution of bromide in hydrogen peroxide and one of bromide in nitrous acid. Both solutions stayed clear, but upon mixing, dibromine was formed as deduced from the reddish-brown color and the smell. He assumed that two hydrogen peroxide molecules reacted with one nitrous acid and proposed the formula HNO4 and the name "Übersalpetersäure"; apparently he was not aware of the publication of Baeyer and Villiger. In 1922, Trifonow explored the properties of the short-lived product of the reaction between nitrous acid and hydrogen peroxide for analytical purposes. He concluded that "naszente Persalpetersäure" (nitric acid in statu nascendi) is capable of oxidizing aniline and nitrating aromatic compounds. He proposed using these reactions for the detection of nitrite and aromatic compounds due to the intensively colored products. In 1929, Gleu and Roell reported that the reaction of azide with ozone results in a deep orange–red solution that smells of hypochlorite and sometimes nitrogen dioxide. Although stable in alkaline solution, the color disappears rapidly upon neutralization by addition of hydrogen carbonate, or by lowering of the pH. In spite of considerable efforts, they were unable to isolate the new unstable compound, but their experiments allowed them to exclude hydrogen peroxide as the oxidant, and they concluded that they were dealing with peroxynitrous acid. Gleu and Hubold described in their paper of 1935 a simple synthesis of peroxynitrite from hydrogen peroxide and nitrite at low pH: one mixes nitrite and hydrogen peroxide, adds acid followed by base within 2 s. If done correctly, the deep yellow color of peroxynitrite is observed. Use of a quenched-flow reactor improves the yield. Kortüm and Finckh showed in 1941 that the absorption maximum of peroxynitrite anion (ONOO-) in the UV ultraviolet range is close to that of NO3-, but the intensity is about 15 times higher and the band is much broader. The initiation of the polymerization of methyl acrylate and the hydroxylation and nitration of aromatic compounds by peroxynitrous acid, reported in 1952 by Halfpenny and Robinson, was rationalized in terms of homolysis of the O–O bond in ONOOH. However, in 1954, Anbar and Taube studied the reactions of peroxynitrite labeled with two O18, and found doubly labeled NO3- as a product of intramolecular rearrangement of peroxynitrite and, in the presence of an excess of unlabeled nitrite, singly labeled nitrite and nitrate as products of O18 transfer from peroxynitrite to nitrite, a result that is not easily explained by homolysis. The first kinetics study of peroxynitrous acid involving its isomerization to NO3- appeared in 1962 and the second in 1969. The latter gives a pKa of 6.6 and a rate of isomerization of 0.10 s-1, obtained at a temperature of 1 °C and an ionic strength of 0.5 M. It also mentioned that peroxynitrite vanishes quickly in the presence of carbonate or borate. Formation of peroxynitrite from nitrate in solution by ultraviolet light was demonstrated in 1964. The same study also showed that permanganate oxidized peroxynitrite. Hughes and Nicklin reported in 1968 the extinction coefficient of peroxynitrite at 302 nm of 1670 [+ or -] 50 M-1 cm-1, which is within the error of that obtained by Bohle and coworkers in 1994 with pure tetramethylammonium peroxynitrite, 1705 [+ or -] 10 M-1 cm-1. Blough and Zafiriou made a very important observation in 1985 of a yellow color after mixing a mostly anaerobic alkaline solution of superoxide with that of nitrogen monoxide. They concluded that superoxide and nitrogen monoxide react to form peroxynitrite. As nitrogen monoxide was identified in 1987 as an "endothelium-derived relaxing factor", and SOD extends the life of nitrogen monoxide, the finding of Blough and Zafiriou could be relevant to physiology!
We see that a few properties and reactions that have been discovered can be used to quantitate peroxynitrite: its yellow color, its ability to nitrate aromatic compounds and the reduction of purple permanganate to green manganate. An excellent review on the "older" chemistry of peroxynitrite by Edwards and Plumb appeared in 1993. It also discusses the role of peroxynitrite in atmospheric chemistry.
From 1901 until 1990, ca. 40 papers on peroxynitrite appeared. Since then, the number of publications has sharply increased to well over 12 000 as a recent search (November 2014) on the Web of Science showed.
1.1.2 1990 and Later
Up to 1990, oxidative injury to tissues was explained with a modification of the Haber–Weiss cycle that included catalysis by iron. In essence, the presence of iron(ii) and hydrogen peroxide was postulated; injury was caused by the Fenton reaction, which, at neutral pH, yields the hydroxyl radical or a higher oxidation state of iron, as reviewed by Koppenol and Bounds. However, Beckman et al. pointed out that "generation of strong oxidants by the iron-catalyzed Haber–Weiss reaction is not an entirely satisfactory explanation for superoxide dismutase (SOD)-inhibitable injury in vivo." Since SOD protects, superoxide must be damaging itself — which it is not — or react to form a more reactive species. Instead of a reaction between some undefined iron(III) complex and superoxide, Beckman et al., referring to the work of Blough and Zafiriou, proposed that superoxide reacts very quickly with nitrogen monoxide to form peroxynitrite. As the reaction in question is a radical–radical reaction, this assumption is quite reasonable. Thus, formation of peroxynitrous acid is kinetically far more feasible than the "iron-catalyzed Haber–Weiss reaction". Beckman et al. also described some scavenger studies to find out whether peroxynitrous acid underwent homolysis to produce nitrogen dioxide and hydroxyl radicals. They concluded that "a potent oxidant similar to HOx in reactivity" was formed. Furthermore, they pointed out that peroxynitrous acid, being long-lived, can diffuse over several cell diameters. For that reason it is a more selective and toxic oxidant than the hydroxyl radical. It is of importance that it also nitrates aromatic compounds. The question of whether nitrogen monoxide and superoxide production under pathological conditions could be high enough to generate peroxynitrite was posed, and we now know that this is so.
First, what are the basics of peroxynitrite chemistry? When it became clear in the early 1990s that peroxynitrite is biologically relevant, we prepared an overview of its thermodynamic and kinetic properties. Much of what we published is still relevant, but some important details have changed. Nevertheless, the paper is still being cited and has now garnered over 1000 citations. Peroxynitrous acid isomerizes with a rate constant of 1.1 s-1. Earlier and slightly higher rate constants have been reviewed. The pKa of peroxynitrous acid is 6.5 to 6.8, depending on the ionic strength and temperature. The peroxynitrite anion is fairly stable, but at pH values equal to the pKa and higher, there is decomposition to nitrite and dioxygen, a reaction that proceeds via an adduct between peroxynitrite and peroxynitrous acid. The spectrum of the anion has a broad maximum at 302 nm (ε = 1705 [+ or -] 10 M-1 cm-1). Peroxynitrous acid is a powerful one- and two-electron oxidizing agent; the calculated one- and two-electrode potentials are, respectively: E°'(ONOOH, H+/NO2x, H2O) = +1.6 [+ or -] 0.1 V and E°'(ONOOH, H+/NO2-, H2O) = +1.3 [+ or -] 0.1 V, both at pH 7. These electrode potentials, as all others, are given relative to the normal hydrogen electrode. Experimentally, a one-electron electrode potential over ca.+1 V was measured by cyclic voltammetry in a cell in which alkaline peroxynitrite was repeatedly mixed with acid to generate the short-lived peroxynitrous acid (t1/2 = 0.63 s at 25 °C). The lack of pH dependence indicates that a transient HOONOx - was formed. It is clear that peroxynitrous acid is a potent one-electron oxidant. The notion that 30% of peroxynitrous acid undergoes homolysis to nitrogen dioxide and the hydroxyl radical is widespread in the literature. We reviewed all evidence, pro and contra, extensively with the conclusion that, if any, homolysis is limited to at most 5%. It may be appropriate to illustrate this point with an example. Above, decomposition to nitrite and dioxygen was mentioned. One can, in principle, explain these products by two homolyses:
ONOOH [arrow right] NO2x + HOx (1.1)
ONOO- [arrow right] NOx + O2x- (1.2)
followed by:
NOx + NO2x [arrow right] N2O3 (+ H2O) [arrow right] 2NO2- + 2H+ (1.3)
HOx + H+ + O2x- [arrow right] H2O + O2 (1.4)
If this is so, then the rate of nitrite and dioxygen formation is limited by the slowest process, which is the reaction in eqn (1.2), that is, it will not depend on the concentration of peroxynitrite. The rate constant of the reaction in eqn (1.2), as determined by the reduction of permanganate by superoxide, is 0.020 s-1. Furthermore, the relative yield of nitrite and dioxygen should also not depend on the peroxynitrite concentration. However, experimentally, we found that both the rate and the relative yield increase with the peroxynitrite concentration. Furthermore, we showed that peroxynitrate is an intermediate:
ONOOH + ONOO- [arrow right] O2NOO- + NO2- + H+ (1.5)
which itself decomposes:
O2NOO- [arrow right] NO2- + O2 (1.6)
Thus, we concluded that peroxynitrous acid behaves like other peracids. Biochemically far more important is the reaction with carbon dioxide. In 1993, Radi's group rediscovered that peroxynitrite is not stable in the presence of hydrogen carbonate. Two years later, Lymar and Hurst found that the peroxynitrite anion reacts rapidly with dissolved carbon dioxide, which leads to ONOOCO2-, nitrosoperoxycarbonate or 1-carboxylato-2-nitrosodioxidane. This compound itself, or its homolysis products, nitrogen dioxide and trioxidocarbonate(x -) are strongly oxidizing: E°(NO2x/NO2-) = +1.04 V and E°(CO3x-/CO32-) = +1.57 V. Although the extent of homolysis is generally assumed to be ca.30%, we estimated it, using nitrogen monoxide as a scavenger of nitrogen dioxide and trioxidocarbonate(x-), at ca. 4%. Thus, peroxynitrite, itself an oxidant with an electrode potential greater than +1 V, may cause the formation of equally strong, or even stronger, oxidants. Reduction of peroxynitrite on a microelectrode with modified surfaces has been used to detect it in biological samples. In 2001, Amatore and coworkers showed that it is possible to oxidize peroxynitrite at a potential of +0.5 V, quite close to the potential of +0.44 V estimated in 1992. This method has been used to detect and quantify peroxynitrite in single fibroblasts and macrophages. Polymerized hemin on a carbon electrode has also been used to oxidize, and thereby, detect peroxynitrite. Chemiluminescence has been used to detect peroxynitrite as well. This technique is sensitive, but in the case of dichlorodihydrofluorescein and dihydrorhodamine, the reaction is zero-order in the indicator molecule, which detracts from its usefulness. Detection based on fluorescence is often not specific for a particular oxidant, and great care should be taken in the interpretation of results. A direct reaction has been established for weakly fluorescent boronates that become strongly fluorescent after reaction with peroxynitrite. As these compounds also react with hydrogen peroxide, there is again the issue of specificity. It is not important that the reaction of boronate with peroxynitrite is much faster than with hydrogen peroxide; it is the product of the rate constant and concentration that counts. In the following chapters, much more will be said about these techniques.
Tetramethylammonium peroxynitrite crystallizes in the cis-conformation and Raman spectroscopy studies indicate that this is also the conformation in solution.
1.2 Peroxynitrite In Vivo
Can peroxynitrite be formed in vivo? Under most conditions, superoxide is disposed of by Cu,Zn-SOD, present in the cytosol at a concentration of ca. 10 µM. Is that enough to prevent the formation of peroxynitrite? Let us assume that the concentration of nitrogen monoxide is ca. 10 nM. If we compare the products of the rate constant of superoxide with Cu,Zn-SOD and with nitrogen monoxide (kSOD = 2 × 109 M-1 s-1, kNOx = 1.6 × 1010 M-1 s-1, respectively), and the concentrations just mentioned, we get 2 × 104 s-1 and 1.6 × 102 s-1, respectively. Thus only 1% of superoxide ends up as peroxynitrite. However, near activated macrophages the concentration of nitrogen monoxide may be in the micromolar region, say 10 µM, and in that case ca. 90% of all superoxide is converted to peroxynitrite. This calculation is simplistic, but it illustrates why the SOD concentration has to be 5–10 µM. There is another reason why SOD is essential. Although superoxide reacts quite quickly with its protonated form, this reaction is second order in superoxide: the initial phase of the reaction is very fast but the end stage proceeds very slowly, and a concentration of zero superoxide is "never" reached. It is the reaction with SOD, which is first order in both superoxide and in proteins, that finishes off superoxide.
We have now established that formation of peroxynitrite in vivo is kinetically feasible. Evidence for its formation in activated macrophages was detected by its ability to nitrate tyrosine 108 of Cu,Zn-SOD. At that time it was also shown that peroxynitrous acid oxidized thiols and lipids. Furthermore, peroxynitrous acid not only nitrates tyrosine, it also hydroxylates it. In atherosclerotic tissue, nitrotyrosine was detected by immunohistochemistry, which is an important observation as it shows that peroxynitrite plays a role in that disease. Antibodies against nitrotyrosine are now commercially available. Furthermore, nitrotyrosine can be detected by high-performance liquid chromatography. Interestingly, the nitration of tyrosine is a reaction that is zero order in tyrosine, and the yield is low, and only somewhat higher in the presence of carbon dioxide. By contrast, tryptophan is nitrated in a bimolecular reaction, but with a low rate constant. To the best of my knowledge, no immunological detection method for nitrated tryptophan has been developed.
(Continues...)
Excerpted from Peroxynitrite Detection in Biological Media by Serban F. Peteu, Sabine Szunerits, Mekki Bayachou. Copyright © 2016 The Royal Society of Chemistry. Excerpted by permission of The Royal Society of Chemistry.
All rights reserved. No part of this excerpt may be reproduced or reprinted without permission in writing from the publisher.
Excerpts are provided by Dial-A-Book Inc. solely for the personal use of visitors to this web site.