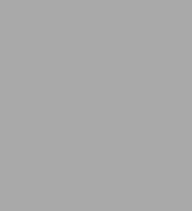
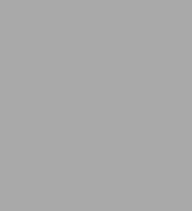
Hardcover
-
PICK UP IN STORECheck Availability at Nearby Stores
Available within 2 business hours
Related collections and offers
Overview
Product Details
ISBN-13: | 9780854048298 |
---|---|
Publisher: | RSC |
Publication date: | 08/09/2006 |
Pages: | 344 |
Product dimensions: | 6.14(w) x 9.21(h) x (d) |
About the Author
Read an Excerpt
An Introduction to Pollution Science
By Roy M. Harrison
The Royal Society of Chemistry
Copyright © 2006 The Royal Society of ChemistryAll rights reserved.
ISBN: 978-0-85404-829-8
CHAPTER 1
Introduction
ROY M. HARRISON
School of Geography, Earth and Environmental Sciences, University of Birmingham, Birmingham, UK
1.1 WHAT IS POLLUTION SCIENCE?
There are various definitions for environmental pollution, all of which contain two key components. The first is that pollution involves some kind of change to the environment. The most obvious kinds of changes are the addition of man-made chemicals that do not occur naturally. Equally important, however, can be the additions of chemicals which do occur naturally in the environment, provided the resultant concentration meets the second criterion. This criterion is that for the phenomenon to be described as pollution, then the perturbation suffered by the environment must in some way be harmful. Not all pollution phenomena are the results of chemicals in the environment. Other important forms of pollution include thermal pollution, an example of which is the discharge of relatively warm power station cooling waters into coastal seas where they can lead to a significant change in the ecology of aquatic organisms. A further example is that of light pollution, caused by the massive amount of urban street lighting, which has a deleterious effect on the environment through obscuring our view of stars in the nighttime sky. Noise pollution has important aesthetic impacts through causing widespread annoyance, but is increasingly suspected of causing adverse effects on health.
This book concerns itself almost exclusively with chemical pollution. It considers the environment as a set of compartments that is familiar to us from our everyday existence. Therefore, separate chapters deal with the atmosphere, the world's waters, and soils and the solid earth. While such a subdivision is convenient in that pollutants behave very differently in each of these media, it is of course not the full story. The atmosphere is very mobile and many pollutants have lifetimes in the atmosphere of only hours or days, although some remain for much longer. Pollutants in the aquatic environment, unless rapidly biodegraded, will often be present for days or weeks, and persistent pollutants for many years. In soils and sediments, however, pollutants can remain relatively immobile for tens or hundreds of years. The rates of mixing are also very different. An atmospheric pollutant with a lifetime of more than a year will become globally mixed, whereas the same degree of mixing throughout the oceans will take centuries and throughout the solid earth will never occur unless there are pathways through water and air. Exchange processes between these major environmental compartments can be very important. Thus, for example, the largest inputs of some pollutants to the North Sea arise through deposition from the atmosphere. There are other examples where pollutants discharged to the sea can become suspended in sea spray and lead to contamination of both the air and the land.
There are two main ways of monitoring chemical pollution. The first and the most obvious is through chemical analysis and that is the main focus of Chapter 5. However, there are also techniques of biological monitoring, which depend upon evaluating the effects of chemical pollution on certain sensitive organisms. Both methods have their advantages and disadvantages. Biological monitoring is rarely specific to a single substance whereas chemical monitoring is. On the other hand, chemical monitoring cannot establish an adverse effect, only a concentration, whereas biological monitoring establishes the effect rather than the concentration. The two are therefore essentially complementary. Chapter 6 examines the ecological and health effects of the chemical pollutants and Chapter 7 the institutional framework for managing the environment.
Pollution science is a relatively new discipline that brings together the various areas of traditional science, mainly from within chemistry, physics and biology, necessary to understand the behaviour of pollutants in the environment, to appreciate their effects on the environment and humans, and to monitor and manage those pollutants.
1.2 THE CHEMICALS OF INTEREST
A very wide range of chemical substances are considered in this book. They fall into three main categories:
(a) Chemicals of concern because of their human toxicity. Some metals such as lead, cadmium and mercury are well known for their adverse effects on human health at high levels of exposure. These metals have no known essential role in the human body and therefore exposures can be divided into two categories (see Figure 1). For these non-essential elements, at very low exposures the metals are tolerated with little, if any, adverse effects, but at higher exposures their toxicity is exerted and health consequences are seen. In the case of the socalled essential trace elements (see Figure 1) the human body requires a certain level of the element, and if intakes are too low then deficiency syndrome diseases will result. These can have consequences as severe as those which result from excessive intakes. In between, there is an acceptable range of exposures within which the body is able to regulate an optimum level of the element. Fluoride is an example of a chemical with a very narrow window of optimal exposure. Fluoridation of water supplies is typically at a level of about 1mg L-1. Half of this concentration may still result in deficiency syndrome and weakened teeth, while double this concentration can lead to the start of adverse effects on teeth and bones.
Environmental exposure to chemical carcinogens is very topical despite the minuscule risks associated with many such exposures at typical environmental concentrations. Examples of chemical carcinogens are benzene (largely from vehicle emissions), and polynuclear aromatic hydrocarbons (generated by combustion of fossil fuels). Figure 2 shows the structures of benzene, benzo(a)pyrene (the best known of the carcinogenic polycyclic aromatic hydrocarbons), and 2, 3, 7, 8tetrachlorodibenzodioxin (the most toxic of the chlorinated dioxin group of compounds). Despite great public concern over the emissions of the last compound, the evidence for carcinogenicity in humans is quite limited.
(b) Chemicals, which cause damage to non-human biota but are not believed to harm humans at current levels of exposure. Many elements and compounds come into this category. For example, copper and zinc are essential trace elements for humans and their environmental exposures very rarely present risk to health. These elements are, however, toxic to growing plants and there are regulations limiting their addition to soil in materials such as sewage sludge which are disposed of to the land. Another category of substance for which there is ample evidence of harm to biota, but as yet little, if any hard evidence of impacts on human populations, are the endocrine-disrupting chemicals. These synthetic chemicals mimic natural hormones and can disrupt the reproduction and growth of wildlife species. Thus, for example, bis-tributyl tin oxide (TBTO) interferes with the sexual development of oysters and its use as an anti-fouling paint for inshore vessels is now banned in most parts of the world. A wide range of other chemicals including polychlorinated biphenyls (PCBs), dioxins and many chlorinated species are also believed to have oestrogenic or androgenic potential, although the level of evidence for adverse effects is variable.
(c) Chemicals not directly toxic to humans or other biota at current environmental concentrations, but capable of causing environmental damage. The prime example is the CFCs, which found widespread use precisely because of their stability and low toxicity to humans, but which at parts per trillion levels of concentration are capable of causing major disruption to the chemistry of the stratosphere.
1.3 UNITS OF CONCENTRATION
The concentration units used in environmental pollution are often confusing to the newcomer. Concentrations of pollutants in soils are most usually expressed in mass per unit mass, for example, milligrams of lead per kilogram of soil. Similarly, the concentrations in vegetation are also expressed in mg kg-1 or µg kg-1. In the case of vegetation and soils, it is important to distinguish between wet weight and dry weight concentrations, in other words, whether the kilogram of vegetation or soil is determined before or after drying. Since the moisture content of vegetation can easily exceed 50%, the data can be very sensitive to this correction.
In aquatic systems, concentrations can also be expressed as mass per unit mass and in the oceans some trace constituents are present at concentrations of ng kg-1 or ITLµITLg kg-1. More often, however, the sample sizes are measured by volume and concentrations expressed as mg L-1 are expressed as parts per million (ppm), µg L-1 as parts per billion (ppb) and ng L-1 as parts per trillion (ppt). This is unfortunate as it leads to confusion with the same units used in atmospheric chemistry with a quite different meaning.
Concentrations of trace gases and particles in the atmosphere can be expressed also as mass per unit volume, typically µg m-3. The difficulty with this unit is that it is not independent of temperature and pressure. Thus, as an airmass becomes warmer or colder or changes in pressure, its volume will change, but the mass of the trace gas will not. Therefore, air containing 1µg m-3 of sulfur dioxide at 0°C will contain less than 1 µg m-3 of sulfur dioxide if heated to 25°C. For gases (but not particles) this difficulty is overcome by expressing the concentration of a trace gas as a volume-mixing ratio. Thus, 1cm3 of pure sulfur dioxide dispersed in 1 m3 of polluted air would be described as a concentration of one part per million (ppm). Reference to the gas laws tells us that not only is this one part per 106 by volume, it is also one molecule in 106 molecules and one mole in 106 moles, as well as a partial pressure of 10-6 atmospheres. Additionally, if the temperature and pressure of the airmass change, this affects the trace gas in the same way as the air in which it is contained and the volume-mixing ratio does not change. Thus, ozone in the stratosphere is present in the air at considerably higher mixing ratios than in the lower atmosphere (troposphere), but if the concentrations are expressed in µgm-3 they are little different because of the much lower density of air at stratospheric attitudes. Chemical kineticists often express atmospheric concentrations in molecules per cubic centimetre (molec cm-3), which has the same problem as the mass per unit volume units.
Aquatic concentrations (including rainwater) are often expressed in chemical equivalents (e.g. meq L-1) and airborne concentrations can be expressed in µeq m-3. The useful aspect of this convention is that for charge neutrality:
[summation] anions in equivalents = [summation] cations in equivalents
The amount in chemical equivalents of anion is calculated from:
equivalents = mass (g)/relative molecular mass x charge
and
µeq = mass (µg)/relative molecular mass x charge
CHAPTER 2The Atmosphere
J.A. SALMOND, A.G. CLARKE AND A.S. TOMLIN
2.1 THE GLOBAL ATMOSPHERE
2.1.1 The Structure of the Atmosphere
2.1.1.1 Troposphere and Stratosphere. The atmosphere consists of a mixture of different gases and particles (both liquid and solid). While there is no fixed boundary between the limit of the Earth's atmosphere and space, most of the atmosphere is found in a zone within 100 km above the Earth's surface. In this zone, which is known as the homosphere, the atmosphere consists of a mixture of gases. Nitrogen (78%) and oxygen (21%) are the two dominant gases, and account for 99% of the total mixture. Other important gases are argon, neon, helium, methane, krypton and hydrogen.
The atmosphere also consists of a large number of trace gases. Depending on their toxicity and proximity to the Earth surface, these gases, which make up only a tiny percentage of the volume of the Earth's atmosphere, can have a disproportionate impact on human health and the environment.
The concentration of trace gases is variable in time and space. This means that the concentrations of trace gases in a sample of air taken from any given point in the atmosphere (in time or space) are likely to be different to those measured in a second sample. This is caused by variations in the processes that release the gases (characteristics of the source), chemical processes operating within the atmosphere and changes in the rate at which they are removed from the atmosphere. For example, in the tropics the concentration of water vapour is about 4% while near the South Pole the concentration is likely to be less than 0.00001%. The length of time trace gases spend within the atmosphere is known as the residence time. For example, the mean residence time for water vapour is about 11 days. After this, condensation and precipitation processes will typically result in its removal from the atmospheric system.
The atmosphere can be divided into 5 different categories or layers which each have different properties. These layers are
the troposphere 0–10 km;
the stratosphere 10–50 km;
the mesosphere 50–90 km;
the thermosphere 90–500 km; and
the exosphere >500 km.
The mesosphere, thermosphere and exosphere account for less than 1% of the total mass of the atmosphere, so emphasis in this chapter is placed on the troposphere (90%) and stratosphere (9.5%). The vertical structure of the atmosphere, showing the features that are most relevant to the problems covered in this chapter, is illustrated in Figure 1. The figure shows the stratosphere, troposphere and boundary layer (that layer which is closest to the Earth's surface). The difference between the layers is characterised by changes in temperature and pressure with height.
The variability of conditions found in the troposphere leads to "the weather" as the layman understands it. The depth of the troposphere varies according to the amount of heat released at the Earth surface. For example, near the equator the mean height of the troposphere is 18 km while near the poles it is only 8 km deep. The temperature of the troposphere is determined primarily by heat release from the surface and thus temperatures decrease with height. Since warm air is less dense than cooler air, the air heated at the Earth's surface rises, causing convectional mixing or turbulence. Frictional forces, resulting from contact between the Earth surface and the atmosphere, act to increase turbulent mixing further and as a result the lower layers of the troposphere are typically well mixed during the day. As a result of the evaporation of water from the Earth surface, more than 95% of the total cloud formation and precipitation can be found in the troposphere. Water vapour in the troposphere however has a short residence time of the order of a few days and weeks.
The tropopause marks the boundary between the moist turbulent troposphere and the dry, stable, ozone-rich stratosphere. The stratosphere typically extends 50 km above the tropospause. The stratosphere is relatively cloud-free and considerably less turbulent – hence long distance passenger jets fly at stratospheric altitudes. In the stratosphere the temperature starts to increase again.
The stratosphere is primarily heated by the absorption of UV radiation from the sun by ozone (O3), concentrations of which are highest between 15 and 50 km. This situation of a layer of warmer, less dense air over a layer of cooler, denser air is quite stable. Consequently, air is typically mixed across the tropopause very slowly unless special events such as tropospheric folding occur.
In the troposphere most pollutants have a fairly limited lifetime before they are washed out by rain, removed by reaction or deposited to the ground. However, if pollutants are injected directly into the stratosphere they can remain there for long periods because of slow downward mixing, resulting in noticeable effects over the whole globe. Thus, major volcanic eruptions injecting fine dust into the stratosphere can lead to a reduction in the amount of solar energy reaching the ground for more than a year after the event. Other global problems relating to events in the stratosphere such as the possibility of damage to the ozone layer are discussed later.
2.1.1.2 Atmospheric Circulation. Identifying the patterns of pollutant transportation within the atmosphere is fundamental to understand global and local environmental problems. The main driving forces for the circulation of the atmosphere are the energy received from incident solar radiation and the Earth's rotation. This results in two main types of global winds: meridional circulation patterns, which transport air north and south, and jet streams, which transport air primarily east to west around the globe.
Due to the angle between the sun and the Earth surface, the amount of solar energy falling on a given area varies with latitude so that the poles are cold and the equatorial regions warm. Warm air rises at the equator and flows North and South towards the poles. At about 30° North and South the air becomes cooler than the surrounding air and starts to sink. The resulting pressure gradient sets up a weak return flow near the surface as air moves from areas of high pressure in the so-called "horse latitudes" back towards the lower pressure areas near the surface at the equator. A similar situation occurs at the Poles where cool air sinks from aloft towards the surface resulting in high pressure at the surface and air moves along the surface towards the polar front located in the mid-latitudes (50–60°). This results in a second rotational cell in each hemisphere. The jet streams (bands of fast-moving latitudinal air flows aloft) typically occur at the junction between these cells.
(Continues...)
Excerpted from An Introduction to Pollution Science by Roy M. Harrison. Copyright © 2006 The Royal Society of Chemistry. Excerpted by permission of The Royal Society of Chemistry.
All rights reserved. No part of this excerpt may be reproduced or reprinted without permission in writing from the publisher.
Excerpts are provided by Dial-A-Book Inc. solely for the personal use of visitors to this web site.
Table of Contents
Chapter 1: Introduction; Chapter 2: The Atmosphere; Chapter 3: The World's Waters; Chapter 4: Soils and Contaminated Land; Chapter 5: Investigating the Environment; Chapter 6: Ecological and Health Effects of Chemical Pollution; Chapter 7: Environmental Management;What People are Saying About This
Suited to students on degree courses in environmental science and pollution studies at both undergraduate and taught postgraduate levels. Its wider pollution-oriented content will also be of value to chemistry students. Some chapters, such as those on the atmosphere, investigating the environment and ecological and health effects of chemical pollution, have been modified and updated. Others, such as the chapters on the world's waters, which replaces separate chapters on the oceans and freshwater, and soils and land contamination, have been written by new authors. Each chapter contains case studies anad is now extensively referenced.