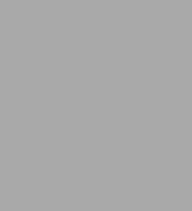
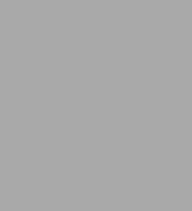
Paperback
-
PICK UP IN STORECheck Availability at Nearby Stores
Available within 2 business hours
Related collections and offers
Overview
Product Details
ISBN-13: | 9781780406855 |
---|---|
Publisher: | IWA Publishing |
Publication date: | 06/16/2016 |
Pages: | 156 |
Product dimensions: | 6.12(w) x 9.25(h) x 0.75(d) |
Read an Excerpt
CHAPTER 1
Introduction to rational nano-design for water applications
Renyuan Li and Peng Wang
In the past century, the development in water treatment technologies has made critical contribution to sustaining human society. Bulk water chemistry-based conventional methods, such as adsorption (Ali & Gupta, 2007; Le Cloirec et al. 1997), advanced oxidization (Liu et al. 2007; Vilve et al. 2009), bio-treatment (Lazarova & Manem, 1995; Lettinga et al. 1980), have been widely utilized at industrial scale for providing quality water for societal benefits. At the same time, with the ever-growing human population and also ever-increasing life quality expectation by each individual, the human society has been pushing the water and energy demand to the limit of environment capacity (Hanjra & Qureshi, 2010; Barnham et al. 2006).
The world energy consumption is 15TW nowadays and it is expected to increase to 30TW by 2050 (Zhang & Wang, 2012). Sadly, the global energy consumption is and will be, for the foreseeable future, heavily dominated by burning non-renewable fossil fuels, especially coal and oil, whose stock is shrinking at an alarming pace and whose usage leads to negative environment impact, majorly environmental pollution and global warming (McCollum et al. 2013) (Figure 1.1). It is true that the water pollution along with water scarcity are becoming severer in many parts of the world as a result of increasing energy consumption by these regions. It is now a popular notion that water security and energy security are two intricately intertwined two grand challenges of our times (Jacobson, 2009; Vorosmarty et al. 2010; Grey & Sadoff, 2007), with neither of which can be solved without looking at the other.
The design of energy and water systems based on bulk chemistry experienced its golden age in the first half of 20 century and has gradually reached their steady states, which propelled research attentions to smaller scales then to beat science hard to show its best to meet the human demand. The concept of 'nano' came into being naturally then. In 1959, Richard Feynman first used the term "nanotechnology" in his famous lecture entitled "there's plenty of room at the bottom", which is hailed by many as the herald of the era of nano (Feynman, 1992).
Nanomaterials have two primary advantages over conventional bulk materials: (1) they have small size and thus big specific surface area, which are beneficial to many interface-related applications; (2) their properties, including chemical, physical, optical, electronic, mechanical, and magnetic properties, can be judiciously tuned by controlling their size, surface morphology, shape and crystal orientation, etc. As a result, going to nanoscale has opened up numerous new avenues that would otherwise be impossible with conventional bulk materials. With a loose definition of nanomaterial being the ones with controllable features at nanometer scale, the general field of nanomaterials has been experiencing literally explosive growth especially during the past two decades and the field of nanomaterial for water applications is no exception (Figure 1.2).
Approach wise, the field of nanomaterial for water applications experienced two two distinct development stages: (1) trial-and-error stage where efforts were made in searching suitable applications in water treatment for the nanomaterials developed by material scientists in a trial-and-error manner. (2) Rational design stage at which nanomaterial design is initiated only based on a scientifically clearly defined problem definition. Within the first stage, water chemists and material scientists tended to work separately and alone and interdisciplinary cross-conversation was rare. By the end of the trial-and-error stage, researchers came to realize that chemistry and ultimately the functions of their nanomaterials could be deliberately pre-designed for a desired purpose. At the rational design stage, the focus is on 'design-for-purpose' before embarking nanomaterial synthesis (Figure 1.3).
In more details, unlike the trial-and-error approach, a rational design process starts with scientifically, generally chemically, defining the problem to be solved. Based on the clear problem definition, a conceptual design of a nanomaterial-based solution is proposed, fed back to the problem definition for scientific check. The communication is iterated until both the problem definition and nanomaterial design agree well with each other. Next, the conceptually designed nanomaterial is checked against the currently available synthesis capability and can then be synthesized if available. Otherwise, the iteration back to the nanomaterial design will take place until the designed nanomaterial can be successfully synthesized. The performance of the synthesized nanomaterial is then assessed with respect to its design purpose, which has been unambiguously defined in the problem definition step and the iteration back to the nanomaterial design will take place again in the event of an unsatisfactory performance of the nanomaterial.
Due to the space limit, the chapter restrains itself from providing more detailed discussion on the concept of 'rational design of nanomaterial for water treatment' and interested readers are encouraged to consult our recent review article on the topic (Li et al. 2015).
There have been numerous exciting developments in the field, but only selected examples are highlighted in this chapter.
1.1 RATIONAL DESIGN OF MAGNETIC NANOMATERIALS AS ADSORBENTS FOR WATER TREATMENT
Adsorption has long been developed as one of well-established water treatment methods (Lambert et al. 1996; Namasivayam & Ranganathan, 1995; Namasivayam & Kavitha, 2002). Designing of an outstanding adsorbent should consider its adsorption capacity, selectivity, stability, reusability, recoverability, and economic feasibility. The development of nanoporous, especially well-ordered mesoporous, materials represents a significant milestone in adsorption as these nanoporous adsorbents possess very high surface areas, large and regularly ordered mesoscale channels, and fast mass transfer kinetics (Wan et al. 2008; Zhang et al. 2009; Yang et al. 2014).
Another important factor influencing adsorbent performance is the interaction between the active sites on the adsorbents and the targeted adsorbates, which is obtained by surface chemical modification of the adsorbents (Zhao & Lu, 1998). The surface chemistry controls the selectivity and strength of the adsorption. Fortunately, many of the nanoporous adsorbents are compatible with a wide range of chemical modifications. As a result, surface chemical functionalization especially of nanoporous materials is a basis of many rational designs of effective adsorbents for water treatment (Walcarius & Mercier, 2010; Feng et al. 1997).
In practical applications, separation and subsequent recycling of the adsorbents is essential from the operation cost point of view. Filtration, centrifugation, sedimentation are among the conventional separation approaches (Wang et al. 2009). Recently, magnetic field induced separation of magnetic adsorbents are emerging as a cost-effective separation method in water treatment (Wang et al. 2010a; Zhang & Kong, 2011).
The advantage of utilizing magnetic separation is obvious particularly in water treatment processes because: (1) it has a lower energy consumption than other conventional methods such as centrifugation especially at large scales; (2) it overcomes fouling and clogging typically occurring in filtration processes; (3) kinetic of magnetic separation is controllable by the intensity of external magnetic field and can be much faster than gravity based sedimentation.
In principle, magnetic properties can be endowed to many conventional adsorbents. One example is Magnetic Ion Exchange (MIEX) resin, in which ion-exchange resin is loaded with high content of magnetic iron oxide particles. Despite the small size (around 180 ?m in diameter), the resin beads provide a large number of adsorption sites and these magnetized resin beads work as weak individual magnets and tend to form agglomerates, which further lead to a rapid settling or fluidize with a high hydraulic loading rates (Singer & Bilyk, 2002). Currently MIEX resins for both anion or cation exchanges are available. Figure 1.4 illustrates an example using MIEX resin for the removal of dissolved organic carbon (DOC) where the adsorption site on MIEX resin is chloride. In regeneration process, the DOC loaded MIEX resin is placed in a concentrated NaCl solution to achieve a reverse ion exchange in which the resin releases DOC and the chloride retakes adsorption site in the resin (MIEX).
Generally, magnetic adsorbents can have two different configurations: (1) magnetic component serving as adsorbent (Ai et al. 2008; Hu et al. 2005); (2) magnetic component providing nothing more than magnetic separation mechanism with the adsorbing component being something else (Yao et al. 2012). The example of the former can be mesoporous γ-Fe2O3 nanoparticles for chromium (VI) removal reported by Wang et al., in which γ-Fe2O3 is both adsorbing component and magnetic component (Wang & Lo, 2009). One example of the latter can be core-shell structured magnetic permanently confined micelle arrays reported by the same group for hydrophobic organic contaminant removal (Wang et al. 2009). The magnetic core of the material does not contribute to the adsorption of the contaminant, but to provide separation means by responding to magnetic field. More detailed discussion on magnetic adsorbents can be found in Chapters 2 and 3.
1.2 RATIONAL DESIGN OF SUPERWETTING MEMBRANE FOR OIL-WATER SEPARATION
The effective and quick removal of accidentally spilled oil in the environment is essential to minimize its adverse environmental impact. Traditional oil spilling responses, including physical skimmers, hydrocyclone based separation, adsorption, face the challenge of low recovery efficiency, high-energy consumption, and high cost (Wang et al. 2015; Zhu et al. 2014). Materials especially membranes based on differentiating surface wetting behaviors between oil, water, have recently attracted considerably research interests (Chu et al. 2015).
For such a material/membrane to be successful, there are many aspects that should be considered into the material design: 1) physic and chemical properties of targeted oil that need be separated from water. 2) Separation material properties, which is essential for the minimization of the challenges such as clogging, fouling and chemical etching. 3) Elongation of membrane or other oil/ water separation material lifetime, such as endowing them with self-cleaning or self-healing properties. As an example, Figure 1.5 shows the concept of a self-cleaning underwater superoleophobic mesh for oil-water separation. Underwater superoleophobic material usually possesses a superhydrophillic surface. When a superhydrophillic material is immersed into water, a water layer forms on the material surface, which prevents its contact with oil. However, once the underwater superoleophobic material is contaminated by oil via adsorption of dissolved species, the material losses its oleophobic property and thus its ability to separation oil/water. To overcome this problem, TiO2 was inducted into the surface coating layer on stainless steel mesh through lay-by-layer assembly method and it endowed the separation mesh a self-cleaning function under the illumination of UV light. The TiO2 helped to decompose the adsorbed fouling species and thus recovered the oleophobicity of the mesh (Zhang et al. 2013) (Figure 1.5).
The Chapter 5 of this book is devoted to rational designing of nanomaterial for controllable oil-separation processes.
1.3 EMERGING NANO-BASED NEXT GENERATION MEMBRANES
The development of conventional membrane based separation, including microfiltration (MF), nanofiltration (NF), ultrafiltration (UF), forward osmosis (FO), reverse osmosis (RO) and membrane distillation (MD), and new nanoassisted membrane processes have recorded a significant progress during past decade (Lee et al. 2011; Hegab & Zou, 2015; Pendergast & Hoek, 2011; Crock et al. 2013). The discovery and development of nanomaterials, often aided by MD simulation nanotechnology open a lot of new avenue beyond the limits of conventional membrane materials (Kalra et al. 2003; Cohen-Tanugi & Grossman, 2012; Walther et al. 2013). The recent rise of two-dimensional nanomaterials especially carbon-based nanomaterials, such as graphene, graphene derivatives, carbon nanotubes, provide the membrane fields with a lot of new possibilities and present a very promising direction in next-generation water treatment.
Alongside with membrane material development, researchers also devote their passion in making synergistically multi-functional all-in-one membranes, many of which represent proof-of-concepts of some ground-breaking and next-generation concepts in water treatment. Comparing with the conventional concept of membranes being permeable and physical barriers for the physical separation of two bulk phases, active filtration is emerging as a more cost-effective alternative wherein adsorption and/or more importantly chemical processes (e.g. catalysis, reduction and oxidization) are being combined with membrane filtration to achieve active filtration for a more energy-efficient water treatment. When operated under a pressure-driven convective flow, the active membranes provide reactants with rapid access to active sites, thereby minimizing the mass transfer limitations associated with other high surface area-to-volume materials and leading to enhanced treatment performance. Examples of active filtration membranes include TiO2 or other semiconductor-based photo (electrochemical)-catalytically active membrane filters (Albu et al. 2007), noble-metal (e.g. Au and Pd) based catalytically active membrane filters (Wang et al. 2013), CNT-based electrochemically active filters (Vecitis et al. 2011) and Fenton-reaction assisted reactive membrane filters (Julbe et al. 2001).
In 2007, Bhattacharyya et al. reported a rationally designed all-in-one Fentonreactionactive filtration system (Figure 1.6), which integrated within one platform nanostructured materials, enzymatic catalysis and iron-catalyzed free radical reactions. Within the fabricated two-layered membrane, glucose oxidase (GOx) was immobilized in the top membrane layer to in situ generate hydrogen peroxide through reacting with deliberately added glucose in the raw water. The as-generated hydrogen peroxide was flushed down to the second layer membrane where it reacted with the polymer-immobilized Fe2+/Fe3+ or iron oxide nanoparticles to initialize Fenton reaction for the oxidization of pollutants in the water within the confined space (Lewis et al. 2011).
The Chapter 4 covers the multifunctional active filtration membranes using hierarchical materials as a design concept.
1.4 RATIONAL DESIGN OF FO DRAW SOLUTION
Unlike reverse osmosis (RO) process, forward osmosis (FO) is a naturally occurring process where water in lower salinity side permeates through a semi-permeable membrane and moves to the side with higher salinity due to the osmosis pressure difference (Yip et al. 2010; Wang et al. 2010b). FO membrane is similar to RO membrane in configuration, with both containing a thin dense layer for salt-water separation and a thick support layer (Lee et al. 2011; Lau et al. 2012). Comparing with RO process which requires a high pressure to overcome natural osmosis pressure to force water molecules through membrane, FO process, due to its require needs no external energy input and has find many niche applications in both water desalination and wastewater treatment (Cath et al. 2006; Zhao et al. 2012) (Figure 1.7).
In parallel to the research efforts in improving FO membrane material's performance, considerable efforts have also been invested in designing novel draw solution to enhance the FO system energy efficiency (McCutcheon et al. 2006; Achilli et al. 2010; Ge et al. 2013). Ideally, a well-designed FO draw solution should generate a high osmotic pressure and lower down the reverse flux of draw solute to enhance the permeate flux and ensure rejection rate. Meanwhile, the draw solution should be easily regenerated after use, and be non-toxic and non-corrosive. One of the commonly used draw solution for FO is inorganic salts, which was first developed in 1970s by Hough et al. (Hough, 1970). The use of salt as draw solution requires further treatment to recover permeate and to regenerate draw solution, which entails additional energy consumption.
(Continues…)
Excerpted from "Rational Design of Next-generation Nanomaterials and Nanodevices for Water Applications"
by .
Copyright © 2016 IWA Publishing.
Excerpted by permission of IWA Publishing.
All rights reserved. No part of this excerpt may be reproduced or reprinted without permission in writing from the publisher.
Excerpts are provided by Dial-A-Book Inc. solely for the personal use of visitors to this web site.
Table of Contents
Editor and contributors, ix,
Preface, xi,
Chapter 1 Introduction to rational nano-design for water applications Renyuan Li and Peng Wang, 1,
Chapter 2 Design and application of magnetic-core composite nano/micro particles for environmental remediation Yuxiong Huang and Arturo A. Keller, 17,
Chapter 3 Rational design of functional nanoporous materials to confine water pollutant in controlled nano-space Swasmi Purwajanti, Jie Yang, Xiaodan Huang, and Chengzhong Yu, 37,
Chapter 4 Hierarchical materials as a design concept for multifunctional membranes Christopher A. Crock, Brian J. Starr, and Volodymyr V. Tarabara, 69,
Chapter 5 Smart membrane materials for controllable oil-water separation Lianbin Zhang and Peng Wang, 81,
Chapter 6 Design of the next-generation FO draw solution Aaron D. Wilson, 103,
Chapter 7 Nanotechnology for microbial fuel cells Muhammad Mustafa Hussain, 131,