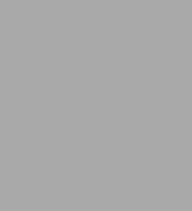
Contemporary Boron Chemistry
552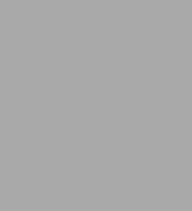
Contemporary Boron Chemistry
552Hardcover
-
SHIP THIS ITEMNot Eligible for Free ShippingPICK UP IN STORECheck Availability at Nearby Stores
Available within 2 business hours
Related collections and offers
Overview
Product Details
ISBN-13: | 9780854048359 |
---|---|
Publisher: | RSC |
Publication date: | 05/26/2000 |
Series: | Special Publications , #253 |
Pages: | 552 |
Product dimensions: | 6.15(w) x 9.20(h) x (d) |
About the Author
Read an Excerpt
Contemporary Boron Chemistry
By Matthew Davidson, Andrew K. Hughes, Todd B. Marder, Ken Wade
The Royal Society of Chemistry
Copyright © 2000 The Royal Society of ChemistryAll rights reserved.
ISBN: 978-0-85404-835-9
CHAPTER 1
Ethylene Oligomerization and Polymerization Catalysts Supported by Boratabenzene Ligands
Zachary J.A. Komon and Guillenno C. Bazan DEPARTMENT OF CHEMISTRY, UNIVERSITY OF CALIFORNIA, SANTA BARBARA, CA 93106, USA
1 INTRODUCTION
The use of the boratabenzene heterocycle as a ligand for transition metal complexes dates back to 1970 with the synthesis of (C5H5B-Ph)CpCo+ (1)(Cp = cyclopentadienyl). Since boratabenzene and Cp are 6 π electron donors, 1 can be considered isoelectronic to cobaltocenium. Many other transition metal compounds have been prepared that take advantage of the relationship between Cp and boratabenzene. In 1996, the synthesis of bis(diisopropy1aminoboratabenzene)zirconium dichloride (C5H5B-NPri2) 2ZrCl2 (2) was reported. Of particular interest is that 2 can be activated with methylaluminoxane (MAO) to produce ethylene polymerization catalysts with activities similar to those characteristic of group 4 metallocenes. Subsequent efforts showed that, under similar reaction conditions, (C5H5B-Ph)2ZrCl2/MAO (3/MAO) gave predominantly 2-alky-l-l-alkenes while (C5H5B-OEt)2 ZrCl2/MAO (4/MAO) produced exclusively 1-alkenes. Therefore, as shown in Scheme 1, it is possible to modulate the specificity of the catalytic species by choice of the exocyclic group on boron.
Scheme 1 raises two important issues. First, relative to standard metallocenes and 2/MAO, the boratabenzene catalysts derived from 3 and 4 show enhanced rates of β-hydride elimination. Secondly, the rates of 1-alkene insertion are considerably faster for 3/MAO relative to 4/MAO. It would be highly desirable to get a deeper understanding of how the electronic and steric effects of different boratabenzene ligands come together to dictate the specificity at the metal. Unfortunately, MAO has a poorly defined and complex structure and is used in large excess relative to the organometallic reagent. Well-defined catalysts that offer the possibility of measuring the rates of individual reactions as a function of boratabenzene ligand should be better study subjects for determining reactivity/structure relationships.
Our working hypothesis for the faster rates of β-hydrogen elimination is that the group 4 boratabenzene catalysts are more electron deficient than their Cp-based counterparts. In other words, even though both Cp and boratabenzene are formally monoanionic 6 π electron donors, boratabenzene is a weaker donor. Further, the orbital overlap between boron and its substituent can be strong and should make an impact on the overall charge density on the boratabenzene ring. As the metal becomes more electron deficient, it interacts more strongly with the β-hydrogens in the growing polymer chain. Agostic interactions of this type have previously been observed in the ground state structures of [CpCo(L)R]+ (L = phosphine or phosphite, R = alkyl)τ Cp*2ScCH2CH38 and (C5H4Me)2Zr(CH2CH2R) (PMe3)+ (R = H, Et, SiMe3, Ph). Such interactions occur when the Cβ-H bond coordinates to an empty metal-centered MO. We propose that in polymerization reactions, as the β-agostic interaction increases in strength, the molecule approaches the transition state for hydride elimination. It is reasonable to expect that for a closely related family of nearly isostructural propagating species, a decrease in electron density should result in faster rates of β-hydride elimination.
Variations in Zr-B bond distances for complexes of the type (C5H5B-R)2ZrCl2 indicate that the nature of R affects the bonding relationship between zirconium and boratabenzene (Table 1). These data imply that for R = Ph, OEt and Me, the interactions between Zr and B are strong. Boratabenzene with R = NPri2 binds in an ν5-pentadienyl fashion with little overlap between B and Zr. Unfortunately, these metrical parameters do not give sufficient insight into differences in the electron density at the metal. Furthermore, they are measurements of precatalyst structures and it is unclear how they relate to the putative cationic catalytic species.
In this contribution we discuss how electrochemical measurements and IR spectroscopy may be used to rank electron donating abilities of boratabenzene ligands. We also try to correlate these measurements with the exchange reactions of well-defined zwitterionic compounds of the type [Cp*(C5H5B-R)ZrMe][MeB(C6F5)3].
2 ELECTROCHEMISTRY
The reduction potential of organometallic species can be used as a probe of the electron density at the metal center. An electron poor metal center is more easily reduced than the corresponding electron rich counterpart. As the ability of a ligand framework to donate electron density increases, the reduction potential of the metal center should therefore become more negative. The use of cyclic voltammetry to measure the reduction potentials of boratabenzene complexes of the type (C5H5B-R)2ZrCl2 (R = NMe2 (5), Oet (4), Me (6), Ph (3)) allows us to rank the boratabenzene ligands according to their donating ability (Table 2). Each of the boratabenzene complexes exhibits a one electron reversible reduction. From this analysis, the observed order of donating ability for (C5H5B-R) is: R = NMe2 > OEt > Me > Ph. The measured value for Cp2ZrCl2 is listed for comparison and shows that Cp is considerably stronger than any boratabenzene studied thus far.
3 CARBONYL STRETCHING FREQUENCIES
It is well established that increased backbonding from the metal to coordinated CO results in a decrease of the CO stretching frequency. The extent of Zr backbonding to CO in complexes of the type (C5H5B–R)2Zr(CO)2 can therefore be used as a relative measure of electron density at zirconium as a function of R.
The dicarbonyl complexes (C5H5B-R)2Zr(CO)2 (R = Ph (7), Oet (8), NMe2 (9), Me (10)) can be easily prepared by reductive carbonylation of the corresponding dichlorides (Scheme 2). FT-IR measurements gave values for the symmetric and asymmetric CO stretches as listed in Table 3. Table 3 also includes the v(CO) values for the mixed ring complexes Cp* (C5H5B–R)Zr(CO)2 (R = Ph (11), OEt (12), NMe2 (13), Me (14)) which were prepared from reduction of the Cp* (C5H5B- R)ZrCl2 precursors under an atmosphere of CO. According to these data the ordering of donating ability of (C5H5B–R) ligands is: R = NMe2 > Me > OEt ~ Ph. The Cp* ligand is a considerably stronger donor and it is useful to point out here that all Cp* (C5H5B- R)ZrCl2/MAO combinations give high molecular weight polyethylene under experimental conditions similar to those in Scheme 1.
4 ZWITTERIONIC SPECIES
The reaction of Cp* (C5H5B-R)ZrMe2 (R = NMe2 (15), Ph (16)) with one equivalent of B(C6F5)3 affords the zwitterionic complexes [Cp* (C5H5B–R)ZrMe][MeB(C6F5]3 (R = NMe2 [(17) Ph (18)) (Scheme 3).
The crystal structures of the zwitterionic species 17 and 18 have been obtained and manifest metrical distortions similar to those of the precatalysts 2 and 3. Compounds 17 and 2 both exhibit long B-Zr distances (2.889(4) Å and 2.98 respectively) as well as shortened N-B bond lengths (1.396(7) Å for both), indicative of B=N double bond character. The structure of 18 displays a shorter Zr-B bond length of 2.770(2) Å.
The variable temperature 1H NMR spectra of 17 and 18 indicate exchange events similar to those observed by Marks and coworkers for standard metallocenes. In this equilibrium process (Scheme 4), the enantiomeric species represented by I interconvert via a solvent separated ion-pair dissociation/recombination process. It is typically assumed that the highest energy species corresponds to structure II.
The activation parameters for the exchange reactions of 17 and 18 were determined by a combination of variable-temperature 1H NMR lineshape analysis and spin saturation transfer experiments. Rate data for 17 were measured over a temperature range of 100 °C. Rates for compound 18 were measured over a 65°C range. The enthalpy of activation was found to be considerably smaller in the case of 17 (12.2(2) kcal/mol) relative to 18 (17.6(3) kcal/mol), Ion pair dissociation is therefore facilitated by the presence of a lone pair of electrons on the boron substituent. The entropy of activation for 17 is -2.3(6) eu, while that of 18 is 8(1) eu. The more positive entropy of activation measured for 18 may be interpreted as the creation of two independent particles from a closely associated ion pair.
5 DISCUSSION
Electrochemical experiments and IR spectra are clearly consistent with the boratabenzene ligand being a stronger donor when the boron substituent is a dialkylamine group instead of phenyl. The data do not allow a similar unambiguous assessment for the intermediate examples of ethoxy and methyl groups. It is likely that steric factors dictate differences in the reactivity of catalysts containing alkoxy- and allcyl-boratabenzene ligands since their electronic properties are so similar." Ligands based on the Cp framework are considerably stronger donors than boratabemne derivatives.
The zwitterionic complexes 17 and 18 behave in the expected fashion. In particular, the "typical" boratabenzene distortions observed with neutral zirconium complexes are retained. The exchange process, which requires generation of the ion separated intermediate II in Scheme 4, occurs more readily for R = Nme2 than for R = Ph. We attribute the faster rates to better stabilization of the positive charge at zirconium by the more donating aminoboratabenzene ligand. These studies show that the role of the boron substituent in controlling the reactivity at the metal is an inherent feature in boratabenzene complexes.
Less is known about the catalysts formed using MAO. The complex structure of MA0 and the large excess that is required for activation do not allow for a careful structure/reactivity correlation similar to that done for 17 and 18. The effect of ligand structure on the selectivities shown in Scheme 1 remain to be understood. We also note that AlMe3 forms an adduct with (C5H5B-OEt) Cp*ZrCl2via the oxygen atom (Scheme 5). Formation of (C5H5B-Me)Cp*ZrCl2 ensues after sufficient reaction time. These observations are significant since commercially available MAO can contain up to 35% of (AlMe3)2 by weight. The simplicity of structure makes complexes of general composition [Cp* (C5H5B–R)ZrMe][MeB(C6F5)3] better candidates for more precise and rational catalyst design.
CHAPTER 2Borate, Boryl and Borole Compounds and their Role in Olefin Polymerisation Catalysis
Manfred Bochmann, Simon J. Lancaster, Gerard Jiménez, Dennis A. Walker, Sarah Al-Benna and Mark Thornton-Pett
SCHOOL OF CHEMISTRY, UNIVERSITY OF LEEDS, LEEDS LS2 9JT, UK
Tris(pentafluorophenyl)borane, a compound first made by Stone et al. in 1963, has in recent years become one of the most widely applied boron reagents, as a highly effective activator for metallocene-based olefin polymerisation catalysts. There are several reasons for this: it is a strong Lewis acid, unlike boron trihalides it is resistant to hydrolysis, and it possesses strong B-C and C-F bonds which make it essentially inert to chemical attack; for example, it can be recovered unchanged from neat elemental bromine.
Due to its Lewis acidity it reacts readily with early transition metal alkyls to give electronically unsaturated, catalytically active species: e.g.:
The Lewis acidity of B(C6F5)3 is also apparent in the ready formation of acidic hydrates. The structure of the monohydrate, obtained by recrystallisation from Et2O containing traces of moisture, is shown in Fig. 1. It contains one hydrogen-bonded EtO molecule.
1. Boryl-Cp Complexes
The active species in B(C6F5)3-activated metallocene catalysts is an ion pair, consisting of an electron-deficient cation, such as [Cp2ZrMe]+, stabilised by a weakly coordinating anion, here [MeB(C6F5)3]-. One aspect of our research recently has been the attempt of building the Lewis acidic activator function into the metallocene precursor complex, in an effort to synthesise "self-activating" systems. The principle is illustrated by the synthesis of the boryl-Cp complex 1. On treatment with AlMe3, 1 readily polymerises ethene, even at low temperatures (-20°C), while CpTiCl3/AlMe3, mixtures are inactive under these conditions. Although the only identifiable organometallic product in this mixture is 2a, the catalytic activity can only be explained by assuming an equilibrium between 2a and the zwitterionic complex 2b which possesses the vacant coordination site required for ethene bonding and catalysis. The fact that in Scheme 1 the equilibrium with B(C6F5)3 is on the side of the zwitterionic product A, whereas in Scheme 2 the neutral dialkyl [2a predominates, illustrates the reduction in Lewis acidity on replacing B(C6F5)3 with CP-B(C6F5)2. A similar reduction in Lewis acidity of borane activators with decreasing electron-withdrawing character of an aryl substituent has recently been found in the series Ar-B(C6F5)2, Ar = 3,5-F2C6H3 > Ph > 3,5-Me2C6H3.
The reaction of 1 with 1 equivalent of LiNHBut gives [CpBTiCl(μ-NBut]2 (3) as red crystals. There is no interaction between the imino-nitrogen and boron, as evidenced by the 11B NMR chemical shift of 3 (6 59.9) which is almost identical to that of 1, (δ 858.9) and B(C6F5)3 (δ 60.1, CDCl3). By contrast, the reaction of 1 with 2 equivalents of LiNHBut affords [CpBTiCl(μ-NBut) (H2NBut]2, 4), where each boron forms an adduct with ButNH2 (11B: δ - 5.0) (equ. 1). The structure of 4 was confirmed by X-ray diffraction
Different structural motifs are found when 1 is reacted with LiCp or LiInd, to give (CpB)CpTiCl2, (5) and (CpB)(Ind)TiCl2. (6), respectively. The spectroscopic data of these complexes are as expected for titanocene dichlorides, except for the 11B NMR resonances which occur at δ 5 ppm, a value indicative of four-coordinate rather than trigonal boron. The assumption of adduct formation is confirmed by the crystal structure of 6 which shows a B-Cl-Ti bridge. The B-Cl bond is relatively short, 2.007(4) Å, and comparable to the terminal B-Cl bond in [ClB(C6F5)3] [1.907(8). Å], whereas the corresponding Ti-Cl(1) bond of 2.4641(9) Å is ca. 6% longer than the terminal Ti-Cl(2) bond of 2.3227( 10) Å.
Repeated crystallisation attempts of 5 eventually gave crystals of the μ-OH complex 7 which shows a similar bonding pattern, with a short B-0 [1.532(3) Å] and a relatively long Ti-O bond (Fig. 3). The Ti-O distance of 2.0437(14) Å, is comparable to the n-donor Ti-OH2, distance in [Cp*2Ti(OH)(OH2)]+x2THF, 2.080(5) Å, whereas the Ti-OH bond in this complex is much shorter, 1.853(5) Å. The structures of 6 and 7 are therefore best described as mittexionic chloro- and hydroxyborates, respectively, with heteroatom n-donor coordination to the metal atom, as opposed to a donor adduct of a Ti-X ligand to the boryl substituents.
The formation of these Cp-B-X bridges is evidently a consequence of the introduction of a bulky Cp or Ind ligand which forces the boryl substituent into closer proximity to the chloride or hydroxide ligands. This is for example reflected in the X-Ti-C(1) angles which decrease from 87.2° in 1 to 68.4° in 6 and 63.7° in 7 (X = Cl or O).
(Continues...)
Excerpted from Contemporary Boron Chemistry by Matthew Davidson, Andrew K. Hughes, Todd B. Marder, Ken Wade. Copyright © 2000 The Royal Society of Chemistry. Excerpted by permission of The Royal Society of Chemistry.
All rights reserved. No part of this excerpt may be reproduced or reprinted without permission in writing from the publisher.
Excerpts are provided by Dial-A-Book Inc. solely for the personal use of visitors to this web site.