Cyclic Peptides: From Bioorganic Synthesis to Applications / Edition 1 available in Hardcover, eBook
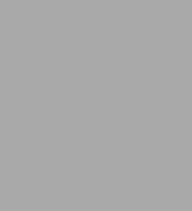
Cyclic Peptides: From Bioorganic Synthesis to Applications / Edition 1
- ISBN-10:
- 1782625283
- ISBN-13:
- 9781782625285
- Pub. Date:
- 12/15/2017
- Publisher:
- RSC
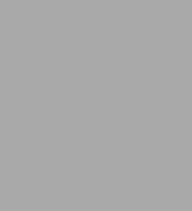
Cyclic Peptides: From Bioorganic Synthesis to Applications / Edition 1
Buy New
$237.00Overview
This book introduces cyclic peptides and provides a thorough overview of biosynthetic and fully synthetic approaches to their preparation. Following an introduction to cyclic peptides, biosynthetic and traditional chemical routes to cyclic peptides are reviewed. Due to their size, their synthesis is not trivial. Recent advances in the incorporation of novel structural units are presented in addition to how synthesis and biological methods can be combined. The chemical analysis of this molecular class is also discussed. Furthermore, chapters detail the progression of cyclic peptides as tools in biology and as potential drugs, providing a future vision of their importance.
In total, this book provides the reader with a comprehensive view of the state-of-the-art of cyclic peptides, from construction to possible clinical utility. This book will be an essential resource for students, researchers and scientists within industry in medicinal, bioorganic, natural product and analytical chemistry fields.
Product Details
ISBN-13: | 9781782625285 |
---|---|
Publisher: | RSC |
Publication date: | 12/15/2017 |
Series: | Chemical Biology , #6 |
Pages: | 392 |
Product dimensions: | 6.15(w) x 9.20(h) x (d) |
About the Author
University of St Andrews, UK
Read an Excerpt
CHAPTER 1
An Introduction to Cyclic Peptides
Martin Empting
1.1 Of Peptides and Proteins (and Small Molecules)
When we compare the Ancient Greek etymologies of 'protein' and 'peptide' it becomes evident that the former of these very closely related biomolecules is associated with rather positive attributes, as its name derives from proteios ([TEXT NOT REPRODUCIBLE IN ASCII.]) meaning "the first quality". Peptides, on the other hand, seem to be considered a mere rudiment of their bigger ancestors, as they are referred to with a terminus derived from peptós ([TEXT NOT REPRODUCIBLE IN ASCII.]), meaning "digested" or "cooked". But what is the decisive characteristic that defines the 'quality' of proteins, which peptides supposedly lack? Both biopolymers usually consist of a linear sequence of amide-linked building blocks, which in most cases are a selection from the standard repertoire of the 20 proteinogenic amino acids. Usually, amino acid sequences shorter than 50 residues are considered as peptides and, thus, these oligomers reside in the so-called 'middle space' (see Figure 1.1).
This term has been coined to refer to molecules with a molecular weight between 500 and 5000 Da (or maybe only approximately 3000 Da). Noteworthily, most of the active principles in pharmaceutical drugs belong either to small molecules or biomedicals. The former usually obey the well-known rules-of-five set up by Lipinski, while the most prominent members of the latter are immunoglobulins or derivatives thereof. Members of the 'middle space' are still comparably rarely found in pharmaceuticals that are in clinical use and small molecular drugs make up about 90% of the pharmaceutical market. However, their share is continuously growing.
So, size as a molecular descriptor might give us a hint toward the 'special quality' of proteins. However, we know of so-called 'mini-proteins' (e.g. McoTI, see Figure 1.1), which can be smaller than 40 residues and exert fascinating biological functions, nonetheless. Hence, certainly, the line between peptides and proteins is fuzzy and not so clear cut as one might think. Which brings us back to the initial question: 'What is the unique quality of proteins, then?'
One or maybe THE extraordinary characteristic of protein chains is their ability to adopt precise spatial arrangements of each individual rotatable bond within their backbone. Only when the huge array of so-called Φ, Ψ and ω dihedral angles is assembled in the right way (see Figure 1.2A), is the protein folded into its correct three-dimensional structure. Partial motifs of protein folds (secondary structures) can also be present in peptides (Figure 1.2B). The most prominent secondary structures are of course α-helices and β-sheets. Aside from larger loops, which are usually not well defined, β-turns should be highlighted as important structural motifs connecting, for example, the separate strands of a β-sheet. However, full tertiary or even quaternary assemblies are usually not found for peptides (Figure 1.2C and D). The reason for this is that a short peptide chain offers fewer opportunities for structure-defining intramolecular interactions, which are needed to render a desired three-dimensional structure thermodynamically favorable. Instead, linear peptides are generally quite flexible and do not adopt an unambiguous geometry. As the structure dictates the function of a biomolecule, we may now have approximated the answer to the question raised above: linear peptides often lack a well-defined structure, while proteins exert their various activities through folding into stable three-dimensional assemblies.
This short repetition of textbook knowledge above instantly raises another question: 'Is there a way to fix a well-defined structure within a peptidic biomolecule?' Well, the title of the book you hold in your hands readily reveals the answer: Yes! – and it can be achieved through introducing cyclic or macrocyclic motifs! Nature and scientists alike make use of these conformational constraints, which drastically reduce the degree of rotational freedom within the backbone, thereby orienting the side chains in favorable directions and, thus, tethering fascinating biological activities and other favorable properties into otherwise inactive peptide sequences.
1.2 Conformational Constraints
Restricting the conformational freedom of a peptide can be achieved by different means. In addition to the just-mentioned macrocyclization strategy, available torsional angles can be reduced through bulky amino acid side chains, where only some of the possible dihedral combinations are allowed at the corresponding and neighboring residues due to steric hindrance. Furthermore, the presence of a proline readily fixes the Φ dihedral at this residue as a consequence of the covalent linkage of the alkyl side chain to the α-amine. The ω dihedral, which is commonly referred to as the peptide bond, can be cemented into each of the two possible conformations through biomimetic exchange, for example by the use of 1,2,3-triazoles or other heteropentacycles. However, introducing a cycle/macrocycle is the strategy that has the most prominent impact on the overall conformational freedom effecting a multitude of residues and not only some selected positions within the peptide chain.
There are three straightforward concepts to achieve a looped structure within a peptide:
Head-to-tail-, side chain-to-side chain-, and side chain-to-terminus-cyclization. Multiple loops are also regularly found in peptides, e.g. a combination of a head-to-tail- and a side chain-to-side chain-macrocycle. If the looped amino acids are only connected via amide bonds, this compound is called 'homodetic'. In cases where any other linkage is involved (e.g. disulfide or depsipeptide bonds), it can be referred to as a 'heterodetic' peptide. According to the IUPAC, a macrocycle consists of at least twelve atoms. Hence the simplest homodetic macrocyclic peptide is a covalent circuit built up of at least four residues. However, smaller (non-macro-)cyclic motifs also occur containing only a limited number of constrained bonds. Figure 1.3 depicts some of the most simple and common motifs. Comparing these structures, it immediately becomes clear that each individual mode of cyclization can result in a completely different geometry of the looped compound.
Noteworthily, far more complex patterns have been observed in peptidic natural products including, for example, thioether linkages commonly found in lanthipeptides. As described for proline, the backbone amide nitrogen can also be used as an anchor point for building a covalent ring structure. Considering all these possibilities for the generation of cyclic peptides, the issue of choosing the appropriate one for each situation is difficult. High-throughput screening methodologies employing e.g. phage display technology can provide access to interesting cyclized peptidic structures. However, researchers often rely on structures that have been provided by nature and can readily be exploited for various applications.
A very prominent showcase for the complexity and finesse of naturally occurring macrocycles is the glycopeptide vancomycin (Figure 1.4). This compound is one of the most important last resort antibiotics for the treatment of multidrug-resistant Staphylococci. Its peptidic portion is rather small, consisting only of seven amino acids.
Interestingly, six of these are non-natural ones with four being d-amino acids. The larger non-peptidic part helps to form the three macrocycles containing a hydroxylated biphenyl motif, an ether-linked dichloroaryl-system and two sugar moieties (glucose and vancosamine).
With its tightly fixed three-dimensional shape, vancomycin is able to selectively bind to d-alanine residues within the peptidoglycan layer of Gram-positive bacteria, thereby disrupting proper cell-wall synthesis and, hence, killing them.
In contrast to the tightly fixed structure of vancomycin, a linear peptide has to adopt a bioactive conformation upon binding to its target. If the flexibility of this compound is high and it can exist in a multitude of different (inactive) geometries, its binding affinity will suffer from a severe entropic penalty significantly affecting the Gibb's free energy of binding (Δ G) and, hence, its binding/dissociation constant (KD) (eqn (1.1) and (1.2)).
ΔG = ΔH - TΔS. (1.1)
with ΔH being the binding enthalpy, T being the temperature, and ΔS being the binding entropy;
[MATHEMATICAL EXPRESSION OMITTED] (1.2)
with R being the universal gas constant.
The establishment of one or maybe multiple constrained cyclic motifs helps to reduce this unfavorable thermodynamic profile of a peptide. Of course, this benefit can only become evident when the applied mode of rigidification does not interfere with binding (e.g. through steric clashes), rendering the rational design of suitable loop structures a difficult, yet, worthwhile endeavor.
1.3 Cyclic Peptides as Pharmaceutical Agents
The number of examples of cyclic peptides from the 'middle space' (vide supra ) as active principles in clinically used drugs is rather sparse compared to the 'classical' approaches relying on small molecular entities or biologicals. To date, around 40 cyclic peptide-based drugs are in clinical use, while the majority of the market is still governed by New Chemical Entities (NCEs, ?90% market share). But the interest of the pharmaceutical industry in developing novel therapeutics based on cyclic peptides is currently growing. For sure, this is a notoriously underexploited structural space with high potential for scientific as well as application-driven exploration.
But first of all, let's have a look at the downsides. In general, peptides are commonly considered as not drug-like. From the perspective of small molecule-based drugs, this holds perfectly true: peptides usually violate the well-known 'Lipinski rule-of-five' used to qualitatively estimate the possible oral bioavailability of a given compound. Even a short sequence of only ten amino acids readily has a molecular weight of over 500 Da, more than five hydrogen bond donors, and more than ten hydrogen bond acceptors, while the high number of rotatable bonds is also supposed to negatively affect oral uptake. Importantly, the amide bonds connecting each of the amino acid monomers are susceptible to enzymatic hydrolysis by proteases and peptidases abundantly present in the intestines, leading to the fast degradation of most peptides. As a consequence, peptides usually possess no or only a very poor oral bioavailability. Hence, the most common routes for administration of peptidic drugs are intravenous, subcutaneous, or intramuscular. However, even if a peptide has entered the bloodstream, it is usually rapidly eliminated from the system by renal clearance. Although from the perspective of small molecular entities peptides are quite large, they are rather small as compared to other biomedicals like immunoglobulin-based drugs. Hence, they fit through the pores of the glomeruli of the kidney, which have a diameter of around 8 nm and effectively remove hydrophilic substances from the plasma. Only larger polypeptides and proteins with a molecular weight cut-off of around 50 to 70 kDa are less prone to elimination by this glomerular ultrafiltration step. Taken together, all these handicaps usually result in a poor pharmacokinetic profile of linear and natural peptides with in vivo half-lives of only a few minutes.
Noteworthily, some of these shortcomings can be overcome by switching the regime from linear to cyclic peptides. First and foremost, it has been well documented that the looped congeners are much more resistant towards hydrolytic degradation. Many peptidolytic enzymes require that the residues at the cleavage site within the peptide substrate accommodate a certain backbone conformation to facilitate proper recognition by the P and P' sites of the protease to promote cleavage of the amide bond. The circular constraint can prevent the compound from adopting this substrate-like conformation and, thus, protect it against the enzymatic attack of exo- and endopeptidases. Of course, the observant reader will notice that the number of rotatable bonds is significantly reduced due to the cyclic constraint as laid out earlier (vide supra), which is an additional benefit. In the case of head-to-tail cyclization, two of the ionizable groups, namely the N- and C-terminus, are removed. This results in a lowered polarity of the compound. Additionally, unfavorable free hydrogen donors and acceptors can be masked due to intramolecular hydrogen bonds made possible by fixing an appropriate geometry and in turn further rigidifying the overall structure. In several cases, it has been demonstrated that cyclic peptides possess improved membrane permeability as compared to their linear variants, which is an important parameter for passive absorption in the gastrointestinal tract. As a consequence, cyclic peptides CAN indeed demonstrate reasonable oral bioavailability. A prime example is cyclosporin A (Figure 1.5), which is obviously 'not drug-like', but has a reasonable bioavailability of about 30%–40% depending on the formulation. It is in clinical use as an immunosuppressive agent for the treatment of e.g. graft-versus-host disease in bone marrow recipients.
Having a closer look at the structure of cyclosporin A, it becomes clear that in addition to the 33-atom-spanning macrocycle, several non-natural amino acids as well as N-methylation at seven of the eleven amide bonds are present. All of these intriguing motifs contribute to the stability of cyclosporin A against enzymatic degradation. In addition to the macrocycle, the extensive N-methylation of this compound has been discussed as another important feature for achieving the unexpectedly good oral bioavailability. The methyl groups reduce the number of unpaired hydrogen bond donors in the molecule, which, as we have learned, should be kept to a minimum. Interestingly, the remaining hydrogen bond donors sum up to a number of five. So at least this Lipinski criterion is fulfilled. As a consequence, masking the most exposed amides through N-methylation has been proposed as a rational design principle to enhance the membrane permeability and, thus, oral bioavailability of a given peptide. Noteworthily, due to the large number of hydrophobic residues and methylated amide nitrogens, cyclosporin A has an undesirable poor solubility. This highlights the necessity to balance the described measures (reduction of hydrogen bond donors and acceptors) against physicochemical parameters during medicinal chemistry optimization.
This has been an encouraging example for a compound with oral bioavailability far beyond the Lipinski space, which fostered further investigations of the possibilities to develop peptide-based drugs for oral intake. From a clinical standpoint, this is highly desirable as the parenteral routes of administration are usually associated with reduced patient compliance due to their invasiveness and inconvenience. Hence, several methodologies, including the generation of pro-drug conjugates or nanoparticular delivery systems, have been studied to improve oral uptake. But a generic approach has not yet been developed and still remains a challenging task. However, applications where oral administration is not the preferred route, like e.g. topical treatment of wounds or pulmonary delivery into infected lungs, can readily make use of cyclic peptides as therapeutic agents.
(Continues…)
Excerpted from "Cyclic Peptides"
by .
Copyright © 2018 The Royal Society of Chemistry.
Excerpted by permission of The Royal Society of Chemistry.
All rights reserved. No part of this excerpt may be reproduced or reprinted without permission in writing from the publisher.
Excerpts are provided by Dial-A-Book Inc. solely for the personal use of visitors to this web site.