Development: The Molecular Genetic Approach
As a result of the molecular genetic analysis of development similar mechanisms for the regulation of gene expression are found in a wide range of organisms. In "Development - the Molecular Genetic Approach" these common mechanisms as well as the specific events leading to a differentiated cell are described. Particular items treated are, for example, how asymmetry is achieved, how cell size is determined, how cell division is controlled, how cell lineage influences development, how cells know their position, and how cells communicate during development.
1116787786
Development: The Molecular Genetic Approach
As a result of the molecular genetic analysis of development similar mechanisms for the regulation of gene expression are found in a wide range of organisms. In "Development - the Molecular Genetic Approach" these common mechanisms as well as the specific events leading to a differentiated cell are described. Particular items treated are, for example, how asymmetry is achieved, how cell size is determined, how cell division is controlled, how cell lineage influences development, how cells know their position, and how cells communicate during development.
54.99
Out Of Stock
5
1
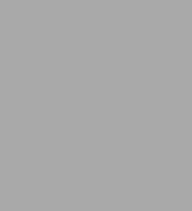
Development: The Molecular Genetic Approach
605
by Vincenzo E.A. Russo, Stuart Brody, David Cove, Sergio Ottolenghi
Vincenzo E.A. Russo
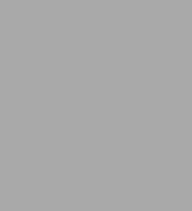
Development: The Molecular Genetic Approach
605
by Vincenzo E.A. Russo, Stuart Brody, David Cove, Sergio Ottolenghi
Vincenzo E.A. Russo
Paperback(Softcover reprint of the original 1st ed. 1992)
$54.99
-
SHIP THIS ITEMTemporarily Out of Stock Online
-
PICK UP IN STORE
Your local store may have stock of this item.
Available within 2 business hours
Related collections and offers
54.99
Out Of Stock
Overview
As a result of the molecular genetic analysis of development similar mechanisms for the regulation of gene expression are found in a wide range of organisms. In "Development - the Molecular Genetic Approach" these common mechanisms as well as the specific events leading to a differentiated cell are described. Particular items treated are, for example, how asymmetry is achieved, how cell size is determined, how cell division is controlled, how cell lineage influences development, how cells know their position, and how cells communicate during development.
Product Details
ISBN-13: | 9783642770456 |
---|---|
Publisher: | Springer Berlin Heidelberg |
Publication date: | 12/05/2011 |
Edition description: | Softcover reprint of the original 1st ed. 1992 |
Pages: | 605 |
Product dimensions: | 7.60(w) x 9.53(h) x (d) |
Table of Contents
Section 1 Microbial Systems, Both Prokaryote and Eukaryote.- 1 Virus Assembly and Morphogenesis.- 1.1 Introduction.- 1.1.1 Viruses as Model Systems for Development.- 1.1.2 Self-Assembling Systems: Molecular Ontogeny?.- 1.1.3 General Principles of Virus Organisation.- 1.2 Molecular Mechanisms of Viral Assembly.- 1.2.1 T4 Bacteriophage, a Complex Virus.- 1.2.1.1 Tobacco Mosaic Virus, a Helical Rod.- 1.2.1.2 Tomato Bushy Stunt Virus, an Icosahedral Shell.- 1.2.1.3 Other Examples: Reovirus.- 1.3 Structural Conservation in Icosahedral Viruses.- 1.3.1 A Conserved Protein Domain.- 1.3.2 Evolutionary Considerations.- 1.4 Outlook.- 1.5 Summary.- References.- 2 Bacillus subtilis Sporulation: a Paradigm for the Spatial and Temporal Control of Gene Expression.- 2.1 Introduction.- 2.1.1 B. subtilis: One of the Best-Known of All Organisms.- 2.1.2 The B. subtilis Life Cycle.- 2.1.3 Advantages and Disadvantages of B. subtilis as an Experimental System.- 2.2 The Powerful Molecular Genetic Approach.- 2.2.1 Defining the Developmental Genes.- 2.2.2 Cloning and Physical Characterization of spo Genes.- 2.2.3 Pathways of Gene Expression.- 2.2.4 Transcriptional Control of spo Gene Expression.- 2.3 Stage 0: Proliferation or Development.- 2.3.1 What Factors Influence the Decision to Initiate Sporulation?.- 2.3.2 The Roles of the spo0 Gene Products.- 2.4 Stages II to III: Generation of Cellular Asymmetry.- 2.4.1 Cellular Asymmetry.- 2.4.2 Differential Gene Expression.- 2.5 Stages IV to V: Differential Morphogenesis.- 2.5.1 Synthesis of the Spore Cortex and Coat Layers.- 2.5.2 Temporal Control of Gene Expression.- 2.6 Outlook and Summary.- References.- 3 Development in Caulobacter crescentus.- 3.1 Introduction.- 3.2 Each Cell Division Cycle Produces a Stalked and a Swarmer Cell.- 3.2.1 Stalked Cell and Swarmer Cell Carry Out Different Programs.- 3.2.2 Methods for Obtaining Synchronous Cell Populations.- 3.2.3 DNA Replication Is Under Cell Cycle Control.- 3.2.4 Membrane Growth and Cell Division.- 3.3 The C. crescentus Flagellum Is Similar to other Bacterial Flagelli.- 3.3.1 The Structure of the Flagellum Is Complex.- 3.3.2 Flagellar Gene Organization.- 3.3.3 Flagellar Assembly Proceeds from the Inside to the Outside.- 3.4 Transcription Controls Timing and Level of Flagellar Gene Expression.- 3.4.1 Alternate Promoters and a Variety of Regulatory Factors.- 3.4.2 Regulatory Hierarchy.- 3.4.3 Timing.- 3.5 Gene Products Are Segregated to the Appropriate Daughter Cell.- 3.5.1 Flagellins.- 3.5.2 Chemotaxis Proteins.- 3.5.3 Pilin.- 3.5.4 Heat-Shock Proteins.- 3.6 Mutations and Antibiotics with Pleiotropic Effects Define Dependent Pathways and Common Developmental Steps.- 3.7 Environmental Influences on C. crescentus.- 3.8 Outlook.- 3.9 Summary.- References.- 4 Streptomyces coelicolor: a Mycelial, Spore-Bearing Prokaryote.- 4.1 Introduction.- 4.2 Physiological Aspects of Early Events in Streptomyces Differentiation.- 4.2.1 Is There an Universal First Signal for Differentiation in Streptomyces?.- 4.2.2 Pheromones and Differentiation in Streptomyces spp..- 4.3 Genes Involved in Streptomyces Differentiation — an Overview.- 4.3.1 The Use of Streptomyces coelicolor for Genetic Studies.- 4.3.2 What Genes are Switched On in Response to the Signals for Initiation of Differentiation?.- 4.4 Development and Metamorphosis of the Aerial Mycelium.- 4.4.1 The Emergence of Aerial Hyphae.- 4.4.2 Overview of Sporulation of Aerial Hyphae and Its Genetic Control in S. coelicolor.- 4.4.3 The Crucial Role of a Transcription Factor in the Onset of Sporulation.- 4.4.4 Not All Sporulation Genes Depend on whiG for Expression.- 4.4.5 RNA Polymerase Diversity in S. coelicolor and Its Relevance to Differentiation.- 4.4.6 The Spore Pigment Locus whiE As a Potential Tool in Analysing Spore Development.- 4.4.7 A Role for Sigma-WhiG in Physiological Regulation?.- 4.5 Implications.- 4.6 Outlook.- 4.7 Summary.- References.- 5 The Programme of Cell Type Determination in Fission Yeast.- 5.1 Introduction.- 5.2 Life Cycle.- 5.2.1 Asexual Life Cycle.- 5.2.2 Sexual Life Cycle: Evidence for Three Cell Types.- 5.3 mat 1 Locus and the Cell types.- 5.4 Mating Type Switching: Homothallism Versus Heterothallism.- 5.5 The Programme of Switching.- 5.6 The Mating Type Region.- 5.7 “Position-Effect” Control for Expression and Switching of Cassettes.- 5.8 Trans-acting switch Genes.- 5.9 The Programme of Switching Is Dictated by Parental DNA Chain Inheritance.- 5.10 Comparison of S. pombe with S. cerevisiae Mating Type Interconversion.- 5.11 Outlook.- 5.12 Summary.- References.- 6 Development in Neurospora crassa.- 6.1 Introduction.- 6.2 Life Cycle.- 6.3 Microbiological and Genetic Techniques.- 6.4 Morphological Studies.- 6.4.1 From Spore to Mycelium.- 6.4.2 From Mycelium to Conidia.- 6.4.3 The Sexual Cycle.- 6.5 Influence of the Environment on Development.- 6.5.1 Environmental Factors Identified So Far.- 6.5.2 The Biological Meaning of Environmental Influence on Development.- 6.6 Developmental Genes of Neurospora.- 6.6.1 Biosynthetic and Developmental Pathways Have Little in Common.- 6.6.2 About the Pleiotropism of Developmentally Relevant Genes.- 6.6.3 On the Role of Mihondria in Development.- 6.7 The Carbohydrate Pathways and Development.- 6.8 Molecular Biology.- 6.8.1 The Mating Type Locus.- 6.8.2 Gene Regulation During Conidiation.- 6.8.3 Blue Light Regulates Many Genes.- 6.9 Outlook.- 6.10 Summary.- References.- 7 Genetic Regulation of Sporulation in the Fungus Aspergillus nidulans.- 7.1 Introduction.- 7.2 Conidiation in the Wild type.- 7.2.1 General Considerations.- 7.2.2 Induction of Conidiation.- 7.2.3 Morphological Alterations.- 7.3 Molecular Genetic Facilities.- 7.3.1 Mutation.- 7.3.2 Complementation.- 7.3.3 Strain Construction and Genetic Mapping.- 7.3.4 DNA-Mediated Transformation.- 7.4 How Many Genes Are Needed for Growth and Development?.- 7.5 Analysis of Development with Stage-Specific Mutants.- 7.5.1 Mutants of Interest.- 7.5.2 Mutants Found but Ignored.- 7.5.3 Mutants Expected but Not Found.- 7.6 Characteristics of Important Developmental Mutants.- 7.6.1 General Considerations.- 7.6.2 Velvet Mutants.- 7.6.3 Fluffy Mutants.- 7.6.4 Aconidial Mutants.- 7.6.5 Bristle Mutants.- 7.6.6 Abacus Mutants.- 7.6.7 Stunted Mutants.- 7.6.8 Medusa Mutants.- 7.6.9 Ivory (Conidiophore Pigment) Mutants.- 7.6.10 Rodletless Mutants.- 7.6.11 Benomyl-Resistant Mutants.- 7.6.12 Wet-White Mutants.- 7.6.13 Spore Color Mutants.- 7.7 Molecular Analysis of Developmental Regulation.- 7.7.1 General Considerations.- 7.7.2 Isolation and Characterization of Regulatory Genes.- 7.7.3 Isolation and Characterization of Responder Genes.- 7.7.4 Controlled Induction of Regulatory Genes.- 7.8 Comparisons with Other Systems.- 7.9 Outlook.- 7.10 Summary.- References.- 8 Development of Trypanosomes.- 8.1 Introduction.- 8.1.1 Why Study Parasites?.- 8.1.2 Trypanosoma and Leishmania Species.- 8.1.3 The Life Cycle of Trypanosoma brucei.- 8.2 Trypanosomes as an Experimental System.- 8.2.1 Trypanosoma and Leishmania — in Vitro and in Vivo Cultivation Are Practical in the Laboratory.- 8.2.2 Energy Metabolism and Respiration in Trypanosoma and Leishmania Species.- 8.2.3 The Nuclei of Trypanosoma and Leishmania Species Have Unusual Properties.- 8.2.4 Trypanosomes Have a Special Mechanism of RNA Processing..- 8.2.5 The Expression of VSG Proteins Is Vital to the Bloodstream Form of African Trypanosomes.- 8.2.6 Differentiation of the Mihondrion of T. brucei Occurs During Development.- 8.2.7 The Mihondrial DNA of Trypanosoma and Leishmania Are Organized into Kinetoplasts.- 8.2.8 kDNA Sequences Participate in RNA Editing.- 8.3 Outlook.- 8.3.1 Mihondrial Development — How Is It Triggered?.- 8.3.2 How Is RNA Editing Developmentally Regulated?.- 8.4 Summary.- References.- 9 Dictyostelium: From Unicellularity to Multicellularity.- 9.1 Introduction.- 9.2 Development of Dictyostelium discoideum.- 9.2.1 Cell-Cell Communication.- 9.2.2 Cell-Cell Adhesion.- 9.2.3 Multicellular Development.- 9.3 Molecular Analysis of Development.- 9.3.1 Cyclic AMP: a Morphoregulatory Signal.- 9.3.2 DIF: a Histospecific Regulatory Signal.- 9.4 Molecular Genetic Analysis of Aggregation.- 9.4.1 Essential Genes in Cell-Cell Communication.- 9.4.2 Cell Adhesion and Motility.- 9.5 Multicellular Development: Determining the Time of Cell Divergence.- 9.5.1 Diffusible Signals, Cell Differentiation and Pattern Formation.- 9.5.2 Cell Adhesion and the Control of Development.- 9.6 Outlook.- 9.7 Summary.- References.- 10 Control of the Cell Cycle in Yeasts.- 10.1 Introduction.- 10.2 Yeast Cell Cycles.- 10.2.1 Cell Cycle Mutants.- 10.2.2 Genes and Clones.- 10.3 Start.- 10.3.1 Pathways in the S. cerevisiae Cell Cycle.- 10.3.2 Start and Commitment.- 10.3.3 Growth Control.- 10.3.4 “Start” in Other Cells?.- 10.4 Coordination of Growth and Division.- 10.4.1 S. cerevisiae.- 10.4.2 S. pombe.- 10.4.3 Size Controls in G1 or G2.- 10.4.4 Size Control in Other Cells.- 10.5 The G2-Mitosis Transition.- 10.5.1 Regulation of the cdc2 Protein Kinase p34.- 10.5.2 MPF and Cyclins.- 10.6 Dependency of Mitosis on Prior DNA Replication.- 10.6.1 S. pombe.- 10.6.2 S. cerevisiae.- 10.7 Pathways in Mitosis.- 10.7.1 Pathways Within Mitosis.- 10.7.2 Dependency of S Phase on Prior Mitosis.- 10.8 Outlook.- 10.9 Summary.- References.- 11 Orcadian Rhythms of Neurospora.- 11.1 Introduction.- 11.1.1 The Neurospora Conidiation Rhythm — an Example of a Synchronized Developmental Event.- 11.2 Input.- 11.2.1 Responses to Light.- 11.2.2 Temperature Effects.- 11.3 The Strategies and Experiments Pursued Towards Understanding the Oscillator.- 11.3.1 The Genetic Approach: Strategies.- 11.3.2 The Biochemical Approach: Strategies.- 11.4 Output.- 11.4.1 Rhythmic Variables.- 11.5 Outlook — Possible Insights into Developmental Events from Studies on the Clock Mechanism.- 11.6 Summary.- References.- Section 2 Plants.- 12 Regulation of Development in the Moss, Physcomitrella patens.- 12.1 Introduction.- 12.1.1 The Suitability of Physcomitrella patens for the Study of Plant Development.- 12.1.2 Development of Physcomitrella patens.- 12.2 Techniques.- 12.2.1 Culture Methods.- 12.2.2 Genetic Methods.- 12.3 The Environmental Modification of Development.- 12.3.1 The Effect of Light.- 12.3.2 The Effect of Nutritional Status.- 12.3.3 The Effect of Phytohormones (Plant Growth Regulators).- 12.4 The Roles of Auxin and Cytokinin in Moss Development.- 12.4.1 Mutants Altered in Hormone Synthesis and Response.- 12.4.2 Continuous Medium Feeding with Auxins and Cytokinins.- 12.5 Tropic Responses.- 12.5.1 Phototropism.- 12.5.2 Gravitropism.- 12.6 Outlook.- 12.7 Summary.- References.- 13 Plant Photoperception: the Phyhrome System.- 13.1 Introduction.- 13.2 Plant Growth and Development Are Responsive to Light Cues.- 13.3 Plant Regulatory Photoreceptors: the UV-B, UV-A/Blue, and Red/Far Red Responsive Systems.- 13.4 Phyhrome Biological Activity.- 13.5 The Molecular Properties of Phyhrome.- 13.6 Photoequilibrium and Regulation of Phyhrome Abundance by Differential Stability.- 13.7 Distribution of Phyhrome.- 13.8 Intracellular Localization of Phyhrome Is Dependent upon Its Conformation.- 13.9 There Are Multiple Types of Phyhrome in Higher Plants.- 13.10 Phyhrome Regulates Plant Gene Expression.- 13.11 Autoregulation of the Phyhrome mRNA.- 13.12 Approaches to the Analysis of Phyhrome Signal Transduction.- 13.13 Outlook.- 13.14 Summary.- References.- 14 Exploration of Agrobacterium tumefaciens.- 14.1 Introduction.- 14.2 Exploration of Agrobacterium tumefaciens.- 14.2.1 Virulence, Function, and Induction.- 14.2.2 T-DNA Transfer.- 14.2.3 Integration of T-DNA.- 14.2.4 T-DNA Expression.- 14.2.5 Host Range.- 14.3 Outlook and Summary.- References.- 15 Exploitation of Agrobacterium tumefaciens.- 15.1 Introduction.- 15.2 T-DNA, a Universal Tool of Plant Molecular Biology.- 15.2.1 Recombination-Based Ti Plasmid Vectors.- 15.2.2 Binary Vectors.- 15.3 Gene Transfer and Transgenic Plant Technology.- 15.4 Gene Expression in Plants.- 15.5 Studying Gene Regulation by Chimeric Plant Promoters.- 15.6 Insertional Mutagenesis: a Link Between Classical and Molecular Plant Genetics.- 15.7 Outlook.- 15.8 Summary.- References.- 16 Developmental Genetics of Arabidopsis.- 16.1 Introduction.- 16.1.1 Biology of Arabidopsis.- 16.2 Genetics in Arabidopsis.- 16.2.1 Classical Genetics.- 16.2.2 Physical and RFLP Maps of Arabidopsis.- 16.3 Molecular Biology of Arabidopsis.- 16.3.1 Genome Size and Organization.- 16.3.2 Gene Transfer in Arabidopsis.- 16.4 Combining Genetics and Molecular Biology to Study Development in Arabidopsis.- 16.4.1 Insertional Mutagenesis Using Agrobacterium.- 16.4.2 Confirmation of Gene Identity by Complementation.- 16.5 Developmental Systems Presently Under Study in Arabidopsis.- 16.5.1 Genetics of Arabidopsis Embryo Development.- 16.5.2 Arabidopsis Phytohormone Mutants.- 16.5.3 Mutants of Arabidopsis That Alter Trichome Development.- 16.5.4 Genetic Analysis of Floral Development in Arabidopsis.- 16.5.5 Development of Roots in Arabidopsis.- 16.6 Outlook.- 16.7 Summary.- References.- 17 Homeotic Genes in the Genetic Control of Flower Morphogenesis in Antirrhinum majus.- 17.1 Introduction.- 17.2 Normal Flower Development.- 17.2.1 General Rules of Normal Flower Development in Higher Plants.- 17.2.2 Determination of Organ Identity During Sequential Organ Initiation.- 17.2.3 Flower Morphogenesis in Antirrhinum majus.- 17.3 Abnormal Flower Development.- 17.3.1 Analysis of Homeosis Can Help to Unravel the Rules Underlying Morphogenesis.- 17.3.2 Three Types of Homeotic Genes Control the Establishment of Flower Organ Identity.- 17.3.3 Differential Induction of Two Pathways Determine Four Organs: a Model.- 17.4 The Role of the deficiens Gene in Floral Organogenesis.- 17.4.1 Morphological Observations on Three Morphoalleles.- 17.4.2 Instability of deficiensglobifera.- 17.4.3 Homeotic Genes Encode Transcription Factors: the MADS-Box.- 17.4.4 Homology Between Floral Homeotic Genes.- 17.4.5 Homeotic Genes Are Upregulated in Two Whorls: Transcriptional Control.- 17.4.6 Posttranslational Processes Specify Homeotic Gene Functions.- 17.5 Outlook.- 17.6 Summary.- References.- 18 The Rhizobium-Legume Symbiosis.- 18.1 Introduction.- 18.1.1 Why Study Nodules?.- 18.2 The Two Symbiotic Partners.- 18.2.1 Rhizobia.- 18.2.2 Rhizobium Genetics.- 18.2.3 Legumes.- 18.2.4 Legume Genetics.- 18.3 Nodule Development.- 18.3.1 Preinfection Events.- 18.3.2 Infection.- 18.3.3 Bacterial Release.- 18.3.4 Nodule Maturation.- 18.3.5 Promoter Structure and Tissue-Specific Gene Expression.- 18.4 Cell to Cell Communication and Gene Expression in Development.- 18.4.1 Cell to Cell Contact.- 18.4.2 Diffusible Signals.- 18.4.3 Physiological Effects.- 18.5 Outlook.- 18.6 Summary.- References.- Section 3 Animals.- 19 Embryogenesis in Caenorhabditis elegans.- 19.1 Introduction.- 19.1.1 The C. elegans Life Cycle Is Rapid and Laboratory Culture Is Simple.- 19.1.2 Each Cell of a Worm Can be Identified Microscopically.- 19.1.3 A Large Number of C. elegans Genes Have Been Identified and Mapped.- 19.1.4 Rapidly Developing Molecular Genetics Provides Tools for Molecular Analysis.- 19.2 An Overview of Embryogenesis.- 19.2.1 Gametogenesis in G elegans Occurs in a Linear Spatial Progression.- 19.2.2 Fertilization Events Designate Anterior-Posterior Polarity.- 19.2.3 Early Cleavages Create Six Founder Cells.- 19.2.4 Further Embryogenesis Consists of Gastrulation, Cell Proliferation, and Morphogenesis.- 19.3 Determination of Cell Fate: Manipulation of Normal Embryos.- 19.3.1 Chromosomal Imprinting Does Not Appear to Affect Cell Fate Determination in C. elegans.- 19.3.2 Cytoskeletal Mechanisms Orient the Spindles Precisely in the Determinative Cleavages of the P Lineage.- 19.3.3 Maternal Cytoplasmic Factors Play a Role in the Determination of Cell Fate.- 19.3.4 Most Lineages Are Established by the 51-Cell Stage but Some Cell-Fate Decisions Depend on Early Cell Interactions.- 19.4 Mutational Analysis of Embryogenesis in C. elegans.- 19.4.1 Maternal Gene Products Control and Support Much of Early Embryogenesis.- 19.4.2 Embryonic Gene Activity Plays a Role in Cell-Fate Determination and Morphogenesis.- 19.5 Outlook.- 19.6 Summary.- References.- 20 Generation of Temporal and Cell Lineage Asymmetry During C. elegans Development.- 20.1 Introduction.- 20.2 unc-86 Couples Cell Lineage and Cell Identity.- 20.3 Genetic Analysis of Cell-Cell Signaling During C. elegans Development.- 20.3.1 let-23 Is Necessary for Intercellular Signaling and is a Member of the EGF-Receptor Superfamily.- 20.3.2 let-60 Is Necessary for Intercellular Signaling and Encodes the G elegans ras homolog.- 20.3.3 Other Genes Involved in Intercellular Signaling.- 20.3.4 Summary of Genes Involved in Cell-Cell Signaling.- 20.4 Genetic and Molecular Studies of Heterochronic Genes in C. elegans.- 20.4.1 A Temporal Gradient in the lin-14 Nuclear Protein.- 20.4.2 How Other Heterochronic Genes Interact with lin-14 to Generate the Temporal Gradient or Interpret It.- 20.4.3 Summary: Generation of the lin-14 Temporal Gradient.- 20.5 Outlook.- References.- 21 Genetic and Molecular Analysis of Early Pattern Formation in Drosophila.- 21.1 Drosophila as a Genetic System.- 21.2 Oogenesis and Embryogenesis.- 21.3 Experimental Embryology.- 21.3.1 The Blastoderm Fate Map.- 21.3.2 Systematic Mutagenesis.- 21.4 The Developmental Gene Hierarchy.- 21.4.1 Anterior-Posterior Determination.- 21.4.2 The Dorso-Ventral System.- 21.5 Outlook.- 21.6 Summary.- References.- 22 Generation of Pattern in Drosophila melanogaster Adult Flies.- 22.1 Introduction.- 22.2 Developmental Analysis.- 22.3 Clonal Analysis.- 22.3.1 Developmental Compartments Are Supra-Cellular Units, Polyclonal in Origin.- 22.3.2 The Elements of the Epidermis Develop in Place.- 22.3.3 Size and Shape of Patterns Do Not Result from Mechanisms Which Count Cell Divisions.- 22.3.4 Cell-Cell Interactions Are Involved in Pattern Formation.- 22.4 Genetic and Molecular Analyses.- 22.4.1 Systemic Genes.- 22.4.2 Cell Communication and Proliferation Genes.- 22.4.3 Terminal Differentiation Genes.- 22.5 Comparative Analysis of Patterns.- 22.6 Outlook and Summary.- References.- 23 Genetic Mechanisms in Early Neurogenesis of Drosophila melanogaster.- 23.1 Introduction.- 23.2 The Neuroectoderm Gives Rise to a Constant Array of Neuroblasts.- 23.3 Cellular Interactions Lead to Cell Commitment.- 23.4 Genes Required for Neurogenesis.- 23.5 The Neurogenic Genes Are Functionally Interrelated.- 23.6 NOTCH and DELTA Encode Transmembrane Proteins with Similarity to the Epidermal Growth Factor, EGF.- 23.7 Physical Interactions Between NOTCH and DELTA.- 23.8 The Enhancer of Split Gene Complex [E(SPL)-C] Comprises Several Related Functions.- 23.9 The AS-C Gene, daughterless and the Genes of the [E(SPL)-C] Are Members of the Same Gene Family.- 23.10 The AS-C Genes Are Required for Neuroblast Commitment.- 23.11 Interactions Between Neurogenic and Proneural Genes.- 23.12 Outlook and Summary.- References.- 24 Xenopus Embryogenesis.- 24.1 Introduction.- 24.2 The Oocyte.- 24.2.1 The Xenopus Oocyte as a Test Tube.- 24.2.2 Nucleocytoplasmic Transport of RNA and Proteins.- 24.2.3 Cytoplasmic Localization of mRNA.- 24.3 Egg Maturation and Fertilization.- 24.3.1 Regulation of Translation During Xenopus Oocyte Maturation.- 24.4 Mesoderm Induction.- 24.4.1 Early Molecular Responses to Mesoderm Induction and the Regulation of Muscle Gene Expression.- 24.4.2 Regulatory Elements in Xenopus Muscle Gene Activation.- 24.5 Gastrulation and Neural Inducation.- 24.5.1 Differential Gene Expression in Neural Induction.- 24.6 Analysis of Gene Function in Xenopus Embryogenesis.- 24.6.1 Inhibition of Gene Expression by Antisense RNA/DNA Techniques.- 24.6.2 Embryo Microinjection of Synthetic mRNA.- 24.6.3 Multiple Families of Nucleic Acid Binding Regulatory Proteins.- 24.7 Outlook.- 24.8 Summary.- References.- 25 The Developmental Regulation of the Genes Coding for 5S Ribosomal RNA in Xenopus laevis.- 25.1 Introduction.- 25.2 Ribosome Biogenesis.- 25.3 DNA.- 25.3.1 The 5S RNA Gene Families.- 25.3.2 The 5S RNA Gene.- 25.4 Transcription: the Active State.- 25.4.1 The Transcription Complex.- 25.4.2 Transcription Factor (TF) IIIA.- 25.4.3 Transcription Complex Stability.- 25.5 Regulation — Establishing Differential Transcriptional Activity.- 25.5.1 The in Vivo Regulation of Differential 5S RNA Gene Transcription.- 25.5.2 The Assembly of Chromatin and 5S RNA Gene Transcription.- 25.5.3 A Model for Differential 5S RNA Gene Expression During Early Development.- 25.6 Regulation — Maintaining the Differentiated State.- 25.6.1 Maitenance of Differential Gene Expression.- 25.6.2 Developmental Regulation of TF IIIA Concentration.- 25.6.3 Multiple TF IIIA Protein Variants.- 25.7 Outlook.- 25.8 Summary.- References.- 26 From Drosophila to Mouse.- 26.1 Introduction.- 26.2 Embryogenesis of the Mouse.- 26.3 Developmental Control Genes Derived from Drosophila.- 26.3.1 Homeobox Genes.- 26.3.2 Engrailed-Type Homeobox Genes.- 26.3.3 Paired-Box Genes.- 26.3.4 Finger-Containing Genes.- 26.4 Octamer-Binding Proteins and Genes.- 26.5 The Protein Products as Transcription Factors.- 26.6 Outlook.- 26.7 Summary.- References.- 27 Cloning Developmental Mutants from the Mouse t Complex.- 27.1 Introduction.- 27.1.1 Why Clone Mouse Developmental Mutants?.- 27.1.2 The Mouse t Complex.- 27.1.3 Historical Perspective.- 27.2 Current Understanding of t Complex Genetics.- 27.3 t Complex-Associated Phenomena.- 27.3.1 Interaction with Brachyury (T).- 27.3.2 Embryonic Lethality.- 27.3.3 Male Sterility and Altered Gamete Transmission Ratios.- 27.3.4 Recombination Suppression.- 27.4 Cloning t Complex Developmental Mutants.- 27.4.1 Step 1: Mapping the Mutant Gene with Respect to Falnking Chromosomal Markers.- 27.4.2 Step 2: Cloning the Genomic DNA Containing the Mutant Gene Locus.- 27.4.3 Step 3: Identifying Coding Sequences in Genomic DNA.- 27.4.4 Step 4: Correlation of a Candidate Gene with the Developmental Mutant.- 27.5 Outlook.- 27.6 Summary.- References.- 28 The Use of in Situ Hybridisation to Study the Molecular Genetics of Mouse Development.- 28.1 Introduction.- 28.2 The Technique of in Situ Hybridisation.- 28.3 The Value of Analysing Expression Patterns.- 28.3.1 Correlation of Gene Expression with Developmental Events.- 28.3.2 Conserved and Divergent Expression Patterns in Different Organisms.- 28.3.3 Genetics and Expression Studies Are Powerful in Combination.- 28.3.4 Expression Patterns Can Provide Insight into Developmental Mechanisms.- 28.4 Some Pitfalls in the Interpretation of Expression Patterns.- 28.5 Outlook.- 28.5.1 Technical Advances in in Situ Hybridisation.- 28.5.2 Insights into Gene Function and Mechanisms of Development.- 28.6 Summary.- References.- 29 Insertional Mutagenesis and Mouse Development.- 29.1 Introduction.- 29.2 Mobile Genetic Elements.- 29.3 Endogenous Retroviruses and Insertional Mutagenesis of the Mouse Germ Line.- 29.3.1 Ecotropic Retroviruses and Insertional Mutation of the Dilute Gene.- 29.3.2 Nonecotropic Retroviruses and Insertional Mutation of the Hairless Gene.- 29.3.3 Spontaneous Reintegrations and Insertional Mutations in Hybrid Mouse Strains.- 29.4 Exogenous Retroviruses and Insertional Activation of Oncogenes in Somatic Cells.- 29.4.1 Identification and Isolation of int-1.- 29.4.2 Identification of Other Proto-Oncogenes.- 29.5 Transgenic Mice and Experimentally Induced Insertional Mutations.- 29.5.1 Identifying and Characterizing New Insertional Mutations.- 29.5.2 Isolating Genes Identified by Random Insertion of Injected DNA.- 29.5.3 Isolating Genes Identified by Random Insertion of Exogenous Retroviruses.- 29.5.4 Inducing and Selecting Specific Insertional Mutations.- 29.6 Outlook.- 29.6.1 New Strategies for Random Insertional Mutagenesis in Transgenic Mice.- 29.6.2 Nonrandom Insertional Mutagenesis: Targeting Mutations by Homologous Recombination.- 29.7 Summary.- References.- 30 The Introduction of Genes into Mouse Embryos and Stem Cells.- 30.1 Introduction.- 30.2 Methods for Introducing Genes into Mouse Embryos.- 30.2.1 DNA Injection into Fertilized Eggs.- 30.2.2 Infection of Embryos with Retroviral Vectors.- 30.2.3 Gene Transfer Using Embryonic Stem (ES) Cells.- 30.3 Expression of Introduced Genes in Transgenic Mice.- 30.3.1 To Study Gene Control.- 30.3.2 To Change the Physiology of Mice.- 30.3.3 To Study Oncogene function.- 30.3.4 To Investigate Complex Systems.- 30.4 The ES Cell System.- 30.4.1 The Gain of Function Approach.- 30.4.2 The Loss of Function Approach.- 30.5 Gene Transfer into Hematopoietic Stem Cells.- 30.5.1 The Hematopoietic System.- 30.5.2 Rationale for Retrovirus-Mediated Gene Transfer.- 30.5.3 Prool for Infection.- 30.5.4 Clonal Analysis of Retrovirus-Transduced Stem Cells.- 30.5.5 Alteration of Hematopoiesis by Retrovirus-Mediated Gene Transfer.- 30.6 Outlook.- 30.7 Summary.- References.- 31 Skeletal Muscle Differentiation.- 31.1 Introduction.- 31.2 Differentiated Skeletal Muscle.- 31.2.1 Physiology and Histology.- 31.2.2 Muscle Diversity and Contractile Protein Isoforms.- 31.2.3 Multigene Families and Alternative RNA Splicing.- 31.2.4 Genetic Studies of Skeletal Muscle Differentiation.- 31.3 Differentiation of Embryonic Skeletal Muscle.- 31.3.1 Transcriptional Regulation During Myoblast Differentiation.- 31.3.2 Identification of Some Myogenic Regulatory Genes: myd and the MyoD Family of Genes.- 31.3.3 Some Properties of MyoD1, myf5, Myogenin, and MRF4 Genes.- 31.4 Embryological Origins of Skeletal Muscle.- 31.4.1 Somite Origins of Vertebrate Muscle.- 31.4.2 Determination of Myoblasts: Role of Cell-Cell Interaction.- 31.4.3 Expression of MyoD in Invertebrates.- 31.5 Outlook.- 31.6 Summary.- References.- 32 Hepayte Differentiation.- 32.1 Introduction.- 32.2 Embryologic Origin of the Liver.- 32.3 Cell Lineages in the Embryonic Liver.- 32.4 Environment Versus Autonomy in Hepayte Differentiation.- 32.5 Cell-Autonomous Genetic Cascades in Hepayte Differentiation.- 32.5.1 Hepayte Nuclear Factor-1?.- 32.5.2 Hepayte Nuclear Factor-1? (HNF-1', also vHNF, vAPF).- 32.5.3 CAAT/Enhancer Binding Protein.- 32.5.4 DBP and LAP, Related Members of the C/EBP Family.- 32.5.5 Hepayte Nuclear Factov-3αβγ (HNF-3αβγ).- 32.5.6 Hepayte Nuclear Factor-4 (HNF-4).- 32.5.7 Combinatorial Models of Liver Determination.- 32.5.8 Combinatorial Models of Transcription Factor Action.- 32.5.9 Multi-Tiered Regulation of Liver Transcription Factors.- 32.6 Environmental Influences on Hepayte Differentiation.- 32.6.1 Circulating Differentiation Factors.- 32.6.2 Cell-Cell Interactions.- 32.6.3 Cell-Matrix Interactions.- 32.7 Outlook.- 32.8 Summary.- References.- 33 Cellular Differentiation in the Hematopoietic System: an Introduction.- 33.1 A Brief Description of the Cells and Their Functions.- 33.1.1 Hematopoietic Stem Cells.- 33.1.2 Progenitor Cells.- 33.1.3 Morphologically Identifiable Immature Cells or Precursors.- 33.2 Shastic Versus Deterministic Mechanisms in the Commitment to Individual Hematopoietic Lineages.- 33.3 Growth Factors in Hematopoiesis.- 33.4 Cell-Cell Contacts in Hematopoiesis.- References.- 34 The Role of Oncogenes in Myeloid Differentiation.- 34.1 Introduction.- 34.1.1 The Normal Myeloid Pathway of Differentiation.- 34.1.2 The Transformed Myeloid Cells to Study Differentiation.- 34.1.3 The Cell Lines as Models to Study Differentiation.- 34.1.4 Retroviruses and Oncogenes.- 34.2 The Role of Oncogenes in Myeloid Differentiation.- 34.2.1 The Avian Defective Retroviruses Which Cause Acute Myeloid Leukemias.- 34.2.2 The Murine Acute Retroviruses Which Transform Myeloid Cells.- 34.2.3 The Murine Chronic Retroviruses that Cause Myeloid Leukemias.- 34.2.4 Potential Oncogenes in Human Myeloid Leukemias.- 34.3 The Role of Proto-Oncogenes in Myeloid Differentiation.- 34.3.1 Studies in Normal Cells.- 34.3.2 Studies of Differentiating Cell Lines.- 34.4. Transgenic Mice.- 34.5 Outlook and Summary.- References.- 35 Developmental Regulation of Human Globin Genes: a Model for Cell Differentiation in the Hematopoietic System.- 35.1 Introduction.- 35.2 The Human Globin Genes: a Paradigm for Cell Type-Specific Gene Expression in the Erythroid Lineage.- 35.2.1 Distant DNA Sequences 5? to the Globin Gene Clusters Regulate the Expression of All Globin Genes.- 35.2.2 Deletions Causing---Thalassemia May Remove a Region Controlling Globin Gene Expression.- 35.2.3 The Locus Control Region (LCR) Allows High-Level, Erythroid-Specific, Position-Independent Gene Expression.- 35.2.4 Crucial Elements of the LCR Are Marked by DNase Hypersensitive Sites (HS Sites).- 35.2.5 LCR Activity May Be Mediated by Long-Range Effects on Chromatin Structure.- 35.2.6 Individual HS Sites Are Sufficient for Position-Independent High-Level-Expression.- 35.3 Erythroid and Stage-Specific Elements Mediating Expression of Individual Globin Genes.- 35.3.1 The Hemoglobin Pattern Is Modulated Throughout the Whole Development Within a Single Cell Population.- 35.3.2 Inherited Defects of the Regulation of Fetal Hemoglobin Synthesis in Humans (Hereditary Persistence of Fetal Hemoglobin, HPFH) Provide Clues to the Identification of Regulatory Elements.- 35.3.3 Transgenic Mice. Globin Gene Regulatory Elements Are Located Both 5?, Within, and 3? to the Genes.- 35.3.4 In Transgenic Mice the LCR Linked to Individual Globin Genes Overrides Their Stage-Specific Regulation.- 35.3.5 Binding Sites for the Same Erythroid-Specific and Ubiquitous Factors Are Present in the Globin Promoter, Enhancer, and LCR Regions.- 35.3.6 GATA-1 and NFE-2 Binding Sites Are Present in Several Erythroid-Specific Genes.- 35.3.7 Elements Regulating Globin Gene Expression Are Conserved in Evolution.- 35.4 Several Hematopoietic Cell Types Express GATA-1.- 35.4.1 Genes Expressed in Megakaryocytes Bind to and Are Regulated by GATA-1.- 35.4.2 The Gene Encoding GATA-1 May Be Activated in a Common Progenitor of Several Lineages.- 35.4.3 GATA-1 Is Necessary, but not Sufficient, for Erythroid Differentiation.- 35.4.4 The Same Transcription Factors Coordinately Regulate Genes Which Are Involved in Several Aspects of Cell Differentiation as Well as Proliferation.- 35.4.5 Which Mechanisms Are Responsible for Establishing the Cell-Specific Pattern of Expression of Transcription Factors?.- 35.5 Growth Factors Are Involved in the Differentiation of Hematopoietic Cells.- 35.5.1 Transfection into Hematopoietic Cells of Genes Encoding Growth Factor Receptors May Stimulate Proliferation and Allow Survival.- 35.5.2 Growth Factors May Cause Differentiation.- 35.6 A Complex Network of Interactions at Several Distinct Levels Underlies Terminal Differentiation.- 35.7 Summary and Outlook.- References.- 36 Growth Control in Animal Cells.- 36.1 Introduction.- 36.2 The Cell Cycle.- 36.3 Controls in the Cell Cycle.- 36.4 Cell-to-Cell Signaling.- 36.5 Growth Stimulatory Factors.- 36.6 Growth Factors and Cell Multiplication.- 36.7 Growth Factors and Transformation.- 36.8 Growth Factor Receptors.- 36.9 The Intracellular Signal Pathway.- 36.10 Oncogenes in the Signal Pathway.- 36.11 Nuclear Response Elements.- 36.12 Cell Cycle-Regulated Genes.- 36.13 Gene Expression at Quiescence.- 36.14 Growth Inhibitory Factors.- 36.14.1 Interferons (IFNs).- 36.14.2 Transforming Growth Factor Beta (TGF?).- 36.15 Control of Growth Arrest.- 36.15.1 Tumor Suppressor Genes.- 36.16 Outlook.- 36.17 Summary.- References.From the B&N Reads Blog
Page 1 of