Extinctions in the History of Life available in Hardcover, Paperback
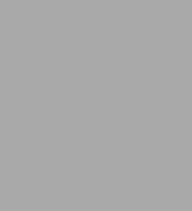
- ISBN-10:
- 0521114896
- ISBN-13:
- 9780521114899
- Pub. Date:
- 06/25/2009
- Publisher:
- Cambridge University Press
- ISBN-10:
- 0521114896
- ISBN-13:
- 9780521114899
- Pub. Date:
- 06/25/2009
- Publisher:
- Cambridge University Press
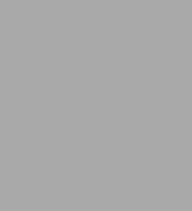
Buy New
$68.99Overview
Product Details
ISBN-13: | 9780521114899 |
---|---|
Publisher: | Cambridge University Press |
Publication date: | 06/25/2009 |
Pages: | 204 |
Product dimensions: | 6.20(w) x 8.90(h) x 0.50(d) |
Read an Excerpt
Cambridge University Press
0521842247 - Extinctions in the History of Life - Edited by Paul D. Taylor
Excerpt
PAUL D. TAYLOR
Department of Palaeontology, The Natural History Museum, London, UK
1
Extinction and the fossil record
INTRODUCTION
The fossil record provides us with a remarkable chronicle of life on Earth. Fossils show how the history of life is characterized by unending change - species originate and become extinct, and clades wax and wane in diversity through the vastness of geological time. One thing is clear - extinction has been just as important as the origination of new species in shaping life's history.
It has been estimated that more than 99 per cent of all species that have ever lived on Earth are now extinct. While species of some prokaryotes may be extremely long-lived (Chapter 2), species of multicellular eukaryotes in the Phanerozoic fossil record commonly become extinct within 10 million years (Ma) of their time of origin, with some surviving for less than a million years. Entire groups of previously dominant animals and plants have succumbed to extinction, epitomized by those stalwarts of popular palaeontology, the dinosaurs. The extinction of dominant clades has had positive as well as negative consequences - extinction removes incumbents and opens the way for other clades to radiate. For example, without the extinction of the incumbent dinosaurs and other 'ruling reptiles' 65 Ma ago, birds and mammals, including humans, would surely not be the dominant terrestrial animals they are today.
Over the past 30 years palaeontologists have increasingly turned their attention towards the documentation of evolutionary patterns and the interpretation of processes responsible for these patterns. As part of this endeavour, extinction has become a major focus of study. Mass extinctions - geological short intervals of time when the Earth's biota was very severely depleted - have received particular attention for two main reasons. First, new geological evidence has been obtained for the causes of mass extinctions (Chapter 5). Second, it has become apparent that the sudden and catastrophic events precipitating mass extinctions have the potential to exterminate species with scant regard for how well they were adapted to normal environmental conditions; the rules of the survival game may change drastically during these times of global catastrophe (Chapter 6). Uniformitarianism, explaining geological phenomena through the action of the slow and gradual processes we can observe in daily operation, has been the guiding paradigm for geologists since Charles Lyell (1797-1875) published Principles of Geology in 1830. However, uniformitarianism alone is insufficient to explain how extinction has moulded the history of life; catastrophic events have also played a key role and this realization is reflected by a revival of scientific interest in catastrophism.
Studies of extinctions in the geological past are relevant in providing a broader context, potentially with remedial lessons, for the contemporary biodiversity crisis being driven largely by the activities of humankind. Published data indicate an accelerating rate of extinction of mammal and bird species for each 50-year interval since 1650. Between 60 and 88 mammal species are thought to have become extinct during the last 500 years, representing about two per cent of the total diversity. Perhaps the most notorious of these extinctions occurred in the late seventeenth century with the disappearance of the Dodo (Raphus cucullatus), a large flightless pigeon from the island of Mauritius in the Indian Ocean, immortalized (in words only) by the phrase 'as dead as a Dodo'. Between 11 and 13 per cent of bird and plant species living today are thought to be close to extinction. A pessimistic estimate considers that up to 50 per cent of the world's biota could face extinction within the next 100 years. Current rates of extinction for relatively well-known groups may be 100 to 1000 times greater than they were during pre-human times (Pimm et al., 1995). Concerns about the human threat to contemporary biodiversity are equally valid for organisms living in the sea as they are for the better known terrestrial biota - the coastal marine environment has been severely disturbed and depleted of diversity by overfishing (Jackson, 2001). While there is no evidence that major extinctions in the geological past resulted from comparable over-exploitation by a single species, there are general lessons to be learnt from ancient extinctions. The most sobering of these lessons is that the Earth's biota recovers extremely slowly after major extinction events. Ten million years or more may elapse before biotas have returned to something like their previous levels of diversity.
This chapter aims to give an introduction to extinctions in the fossil record, setting the scene and providing a general background for the more detailed accounts in the chapters that follow. After a brief historical preface, I describe how extinction is detected and measured in the fossil record, the broad patterns of extinction and biodiversity change that are evident in the Phanerozoic fossil record, and the interpretation of extinction patterns and processes.
BRIEF HISTORY OF FOSSIL EXTINCTION STUDIES
Two hundred years ago there was no general agreement among naturalists that any species had ever become extinct. Although naturalists at that time knew of fossil species that had never been observed alive, most of these were marine animals and it remained possible that they would eventually be discovered living somewhere in the poorly explored seas and oceans of the world. The great French naturalist Georges Cuvier (1769-1832; Figure 1.1A) is generally accredited with establishing the reality of ancient extinctions. Cuvier's work on the fossils from Cenozoic deposits in and around Paris revealed the former existence of several species of large terrestrial mammals ('quadrapeds') not known to be living at the present day but which would surely have been discovered if they had been: 'Since the number of quadrapeds is limited, and most of their species - at least the large ones - are known, there are greater means to check whether fossil bones belong to one of them, or whether they come from a lost species.' (Cuvier, 1812, translation in Rudwick, 1997). Cuvier promoted the idea of catastrophism to explain the extinction of species. According to him, major geological upheavals, unlike anything witnessed by humankind during modern times, were responsible for these extinctions. One of Cuvier's main reasons for favouring catastrophic extinction was his belief that species were so well-adapted that their gradual extinction was inconceivable (Rudwick, 1997).
Alcide d'Orbigny (1802-57; Figure 1.1B), a student of Cuvier's who undertook detailed research on the taxonomy and stratigraphical distribution of fossil invertebrates, extended his mentor's ideas. D'Orbigny's findings led him to propose that life on Earth had been devastated by 27 catastrophic extinctions. All living species were exterminated during each extinction event, subsequently to be replaced by a totally new biota formed in a fresh creation of life. The stratigraphical stages (e.g. Bajocian, Cenomanian) erected by d'Orbigny, still used by geologists
Figure 1.1 Portraits of some pioneers in the early study of fossil extinctions. A, Georges Cuvier; B, Alcide d'Orbigny; C, Charles Darwin; D, John Phillips.
today in a modified way, each represent an interval of geological time when the Earth was populated by one of the 27 biotas. In contrast to the catastrophist creationists Cuvier and d'Orbigny, Charles Darwin (1809-82; Figure 1.1C) was an evolutionist who followed the uniformitarian principles expounded by Lyell. He believed in the gradual disappearance of species, one after the other, rather than their sudden decimation. He considered that natural selection was sufficient to explain the extinction of species, writing in the Origin of Species (1859) that 'the improved and modified descendants of a species will generally cause the extermination of the parent-species.'
The British geologist John Phillips (1800-74; Figure 1.1D) made an early attempt to estimate the broad changes in the diversity of life on Earth between the Cambrian and the present day. Phillips' (1860) plot of diversity against time shows two major drops, one at the end of the Palaeozoic and the second at the end of the Mesozoic. Through his first-hand experience of fossils and their distribution in strata of different ages, Phillips was able to recognize the great turnovers of life that marked the transitions between the Palaeozoic, Mesozoic and Cenozoic eras. The wholescale extinctions of species at these era boundaries we now call the end-Permian and Cretaceous-Tertiary (K-T) mass extinctions (Figure 1.2).
Skipping forward to the final quarter of the twentieth century, the contributions of two research groups ignited a major resurgence of interest in extinctions in the fossil record. Jack Sepkoski's compilation of the ranges through the Phanerozoic of marine families, and later of marine genera, opened the way for the analysis of global extinction patterns undertaken in collaboration with David Raup. A landmark paper (Raup and Sepkoski, 1982) on extinction rates enabled five mass extinctions to be recognized in the Phanerozoic, and a subsequent analysis (Raup and Sepkoski, 1984) suggested a 26-Ma periodicity in extinction between the end of the Permian and the present day. The first of these papers stimulated a flurry of work among palaeontologists interested in how particular taxonomic groups had fared during these 'Big Five' mass extinctions (e.g. Larwood, 1988), while the claim of periodicity prompted various astronomical explanations of causal mechanisms, engagingly summarized by Raup (1986).
At about the same time that Sepkoski and Raup were compiling and analysing data from the fossil record, a team led by Luis and Walter Alvarez at UC Berkeley discovered an enrichment of the element iridium at the K-T boundary in Gubbio, Italy (Alvarez et al., 1980), coincident with the K-T mass extinction which removed the last dinosaurs and many other species. This iridium anomaly, later identified at the same stratigraphical level elsewhere in the world, provided strong evidence for the impact of a sizeable extraterrestrial object (bolide or asteroid) with the Earth, an impact with numerous possible consequences devastating to life on the planet. Initially received with scepticism by most palaeontologists, the impact hypothesis for the K-T mass extinction has since won considerable support. Identification of the apparent impact crater (Chicxulub, Mexico) and a wealth of other
Figure 1.2 Examples of species belonging to marine invertebrate groups that suffered extinction during the end-Permian and end-Cretaceous (K-T) mass extinctions. Note that the species depicted were not among the final survivors of their groups, and that rudists as a whole may have succumbed a little before the K-T event. Original lithographs taken from Nicholson (1879).
geological evidence (shocked quartz grains, tektites, tsunami deposits, etc.) have corroborated the original hypothesis, although the kill mechanism/s and the possible involvement of other environmental changes in the K-T mass extinction are still contentious issues (Chapter 5).
DETECTING AND MEASURING EXTINCTIONS
What exactly is extinction?
Extinction is quite simply the 'death' of a taxon. The extinction of a species occurs when the last individual of that species dies. The extinction of a genus happens when the last individual belonging to the last species of the genus dies, and so on. In the case of a small number of species that have become extinct in historical times, the death of the last individual, and therefore the extinction of the species, has actually been observed. For example, the last Tasmanian tiger (Thylacinus cyanocephalus), a wolf-like marsupial mammal, died in Hobart Zoo on 7 September 1936. Nonetheless, unsubstantiated sightings of Tasmanian tigers in the wild are still occasionally reported, illustrating that even for such a large and distinctive contemporary animal it can be difficult to verify extinction.
Detecting extinction in the incomplete fossil record
Pinpointing the moment of extinction is much more of a problem in the fossil record than it is among organisms that became extinct during historical times. Even large-scale extinctions seldom generate mass mortality deposits (cf. Zinsmeister, 1998) where palaeontologists might expect or hope to find the last individuals belonging to a species. No palaeontologist would ever claim that a particular fossil specimen represents the very last survivor of a species - the probability of this last individual being fossilized, discovered and collected are infinitesimally small. Even if we did have this fossil to hand we could never be sure that this is what it was. A fundamental difficulty with extinction is that it is impossible to prove a negative - the absence of a species - and therefore to be sure exactly when extinction occurred. Nevertheless, repeated interrogation of the fossil record does allow scientists to corroborate and refine assessments of when a species, or a clade, became extinct. We can, for instance, be confident that the last ammonites became extinct at or before the K-T boundary because intensive sampling of younger, post-Cretaceous rocks has failed to produce any unequivocally indigenous ammonites. The last appearance of a species (or genus, family, etc.) in the fossil record seldom coincides with the time of its extinction. Instead, the last appearance will always precede the true time of extinction because of the incompleteness of the fossil record. Stratigraphical completeness sets an upper limit on the completeness of the fossil record - the fossil record can never be more complete than the rocks containing the fossils. Schindel (1982) calculated completeness for seven stratigraphical successions which had been used in evolutionary studies because they were regarded as being relatively complete. Even in these exceptionally complete successions, stratigraphical completeness never exceeded 45 per cent and was 10 per cent or less for five of the seven successions.
Signor and Lipps (1982) realized that sampling gaps in the fossil record could make the severity of a mass extinction event seem less than it actually was. The last appearances of taxa before their true time of extinction are 'smeared' through an interval of time before the mass extinction. This sampling artefact is termed a Signor-Lipps Effect (Figure 1.3). For example, Rampino and Adler (1998) showed how a Signor-Lipps Effect could account for the extinction pattern of foraminifera before the end-Permian mass extinction in the Italian Alps. Species of foraminifera with overall lower abundances through a sequence of Permian rocks are the first to disappear from the fossil record, whereas more abundant species range higher in the section, as would be predicted if their last appearances in the fossil record were determined by sampling.
Another pattern resulting from the incompleteness of the fossil record occurs when a species apparently becomes extinct only to reappear in younger rocks (Figure 1.3). This is known as a Lazarus Effect, after the disciple who reputedly returned from the dead. Taxa missing from the fossil record but which can be inferred to have been alive at the time by their occurrence in both older and younger rocks are called Lazarus taxa (see Fara, 2001). Lazarus taxa are useful in assessing the quality of the fossil record - the greater the proportion of Lazarus taxa present during a given interval of geological time, the poorer is the fossil record for that time interval. Times of apparently high extinction intensity may sometimes be reinterpreted as due to deficiencies in the fossil record when a high proportion of Lazarus taxa are present. However, Lazarus taxa do often increase in number at times of true mass extinction (e.g. Twitchett, 2001) because the same factors that bring about the genuine extinction of some taxa may cause other taxa to shrink in geographical range and/or population size (Wignall
Figure 1.3 Two important patterns for extinction studies caused by gaps in the fossil record illustrated by range data for 12 hypothetical species with respect to an extinction horizon. The backward smearing of last appearances of species that became extinct at a major extinction event is known as a Signor-Lipps Effect. Temporary disappearance of taxa through an interval of time, often associated with true extinction of other taxa, produces a Lazarus Effect.
and Benton, 1999), removing them temporarily from the fossil record until more normal conditions return. Survival of Lazarus taxa is usually explained by postulating the existence of refuges - safe places where the adverse factors causing extinction were absent or reduced. For every extinction event, there are always particular habitats and/or geographical regions not represented in the fossil record that might have provided refuges for Lazarus taxa.
Pseudoextinctions in the fossil record should be distinguished from true extinctions. The subdivision of an evolving lineage into two or more species results in the pseudoextinction of the ancestral species at each transition. It would be incorrect to classify this as a true extinction because genetic continuity is maintained between ancestral and descendant species - the branch on the evolutionary tree is not terminated but continues under a different name. The change of species name is sometimes placed at an arbitrary point within the lineage, for example coinciding with a geological boundary, or a time of particularly rapid morphological change. Alternatively, it may be made at an abrupt morphological jump.
A second kind of pseudoextinction can occur when we deal with taxa above the species level. The pseudoextinction of paraphyletic higher taxa (paraclades) is best illustrated using a frequently cited example, the dinosaurs. Dinosaurs of popular understanding - large, land-dwelling animals such as Brachiosaurus and Tyrannosaurus - are a paraclade. Only when birds are included within dinosaurs do we get a true clade. This is because birds are more closely related to some dinosaurs than these dinosaurs are to other dinosaurs, that is birds and these dinosaurs share a more recent common ancestor. While there is little doubt that the last dinosaur species of popular understanding did become extinct during the K-T mass extinction, birds survived this extinction, carrying their dinosaurian genetic legacy through to the present day. Leaving aside semantic aspects, the importance for extinction pattern analysis of including or excluding pseudoextinctions of paraclades in databases has been debated vigourously by palaeontologists. Until a great many more phylogenetic analyses have been completed, most fossil data on extinction patterns will inevitably comprise a mixture of true clades and paraclades. Simulation studies suggest, however, that inclusion of paraclades does not substantially alter extinction patterns, and even the loss of a paraclade, such as the dinosaurs, involves the true extinction of one or more species.
Measuring extinction
A variety of metrics have been applied to quantify extinction in the fossil record (Figure 1.4). The simplest is number of extinctions (E), i.e. the number of taxa becoming extinct. This measure has limited applicability in studies of extinctions through geological time because it is dependent on the duration of the time interval in question and on the number of taxa present during that interval. Geological time is not conventionally divided into slices of even duration - stratigraphical stages, a commonly used division, differ substantially in their durations. A large value of E may simply reflect a time interval of greater than average length. Therefore, extinction rate (E/t) is often calculated, usually expressed per million years. Another metric - per taxon extinction (E/D) - allows for differences in diversity by dividing the number of extinctions by the diversity (D) of taxa present during the time interval
Figure 1.4 Metrics used to quantify extinction intensity. Range bars for 10 taxa are shown, all present within the shaded stratigraphical interval of interest which has been assigned an arbitrary duration of 2 million years (Ma). Five of the taxa have their last appearances within this interval, i.e. suffer extinction. This is equivalent to a per taxon extinction of 0.5 (or 50 per cent), a rate of extinction of 2.5 taxa per Ma, and a per taxon extinction rate of 0.25 per Ma.
in question. A third commonly used metric is per taxon extinction rate (E/D/t). While compensating for variations in both duration and diversity has clear advantages, errors can be introduced if there are uncertainties in the length of time represented by the interval or in the actual diversity of taxa present. The use of different extinction metrics can sometimes affect perceptions of extinction patterns. For example, the relative severities of bryozoan genus extinction in the terminal stage of the Cretaceous (Maastrichtian) and basal stage of the Tertiary (Danian) change according to the extinction metric used (McKinney and Taylor, 2001).
© Cambridge University Press