Jacobson's Organ: And the Remarkable Nature of Smell available in Paperback, eBook
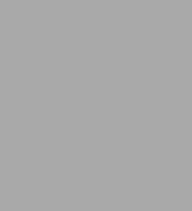
Jacobson's Organ: And the Remarkable Nature of Smell
- ISBN-10:
- 0393332918
- ISBN-13:
- 9780393332919
- Pub. Date:
- 04/17/2000
- Publisher:
- Norton, W. W. & Company, Inc.
- ISBN-10:
- 0393332918
- ISBN-13:
- 9780393332919
- Pub. Date:
- 04/17/2000
- Publisher:
- Norton, W. W. & Company, Inc.
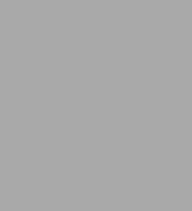
Jacobson's Organ: And the Remarkable Nature of Smell
Buy New
$21.95Overview
Smell is our most seductive and provocative sense, invading every domain of our lives. We can identify our relatives, detect the availability of a potential mate, sniff out danger, and distinguish between good and bad food just with our noses. In this surprising and delightful book, Lyall Watson rescues our most unappreciated sense from obscurity. He brings to light new evidence concerning Jacobson's Organ: an anatomical feature discovered high in the nose in 1811 and dismissed for centuries as a vestigial ghost. Yet recent research has shown Jacobson's Organ to be an incredibly influential pheromonal mechanism that feeds the area of the brain affecting our awareness, emotional states, and sexual behavior.
Following the seven classes of smell devised by the pioneering botanist Carl Linnaeus in his Odores Medicamentorum, Watson examines the roles of smell and pheromones in humans, plants, and animals. He reveals the curious ways in which trees communicate their distress, the olfactory abilities of feral children, the bond we have with our offspring, the psychosexual effects of perfume, and the link between smell and memory formation. Jacobson's Organ unlocks the door to the strange world of this mysterious sense.
Product Details
ISBN-13: | 9780393332919 |
---|---|
Publisher: | Norton, W. W. & Company, Inc. |
Publication date: | 04/17/2000 |
Pages: | 268 |
Product dimensions: | 5.00(w) x 8.00(h) x (d) |
About the Author
Read an Excerpt
Chapter One
A nose is born
Smell was our first sense. It is even possible that being able to smell was the stimulus that took a primitive fish and turned a small lump of olfactory tissue on its nerve cord into a brain. We think because we smelled.
The argument is simple. Before sight and sound hijacked our attention, we shared with all life a sort of common sense, a chemical sense that depended on direct contact with matter in the water or the air. And for 90 per cent of our time on Earth, that was how things worked.
Then the emphasis shifted. We learned to live instead with waves of energy, making sense of chaos, and becoming conscious. Which, of course, was a good thing ... but we need now to go back and pick up some useful talents that we abandoned on the way.
Four hundred million years ago, in the Devonian period, fish were the most important and advanced animals on Earth. And the most abundant of them were jawless, heavily armoured forms, filtering their food out of thick coastal muds. Theirs was a world of direct sensation. Behaviour was simple. Any unusual stimulus, a bright light, a loud sound, any abrupt contact, produced much the same response: one of aversion. They pulled back and tried again later, taking life one taste at a time.
In the beginning, it was difficult to separate taste and smell. In the mud, sensation followed contact. You bumped into things and then tested them to see whether they were worth avoiding or eating. The tests were chemical ones, made by cells designed toanalyse molecules dissolved in the water.
Many crustaceans still work this way, using cells on their legs which respond only to amino acids, providing a ready distinction between organic and inorganic matter. Filter-feeding fish didn't need to be much more discriminating, extracting what was useful from the mud and rejecting the rest. But instead of just oozing around everywhere with their jawless mouths wide open, it would have helped these mindless pioneers to have some way of finding the most nutritious muds. What they needed was the ability to test and taste from afar and that is where smell comes in.
Smell is a long-distance sense, a way of stretching time and finding out in advance what lies ahead. It expands both awareness and opportunity, providing a need for analysis that normally never touches the lives of bottom feeders. But it was, in fact, one of those mudsuckers that made the big leap. And the result is visible still, frozen in the life histories of a few modern species of jawless, limbless, boneless and often blind hagfish and lampreys.
Hagfish are patient scavengers, spending most of their lives embedded in soft mud with only their blunt snouts protruding, waiting for the same chemical signal that attracts crustaceans to putrid fish. But these primitive fish have an advantage over lobsters and shrimps. They have a single opening above the mouth that leads to a paired chamber in which scents can be isolated, analysed and perhaps even located. They have, it seems, the world's first noses.
Armed with these secret weapons, hagfish have flourished. At least twenty species survive, some so well that they have become a nuisance to modern fishermen, burrowing into catches of haddock and cod and consuming netted fish from the inside out, turning them into bags of bones. Hagfish see nothing, but clearly smell very well, swimming up a gradient of fishy flavours, travelling with undulating movements, turning always in the direction of the strongest stimulus, choosing the right odour corridors, keeping on going until they get there.
Their relatives the lampreys have refined the process even further, responding instinctively to just one aromatic chemical that forms part of the normal body odour of live schooling fish such as trout. Sensing this, they left their muddy larval haunts, developed functional eyes, and now actively pursue and parasitize such hosts. Once within range, the swift eel-like lampreys use their rasping teeth to hold on to any soft part of a trout, sucking out its body fluids like aquatic vampires, keeping their victims' blood flowing just as bats do, with anticoagulants in their saliva.
The unpleasant habits of round-mouthed hagfish and lampreys are vividly described as 'suctorial'. Theirs is a mode of life made possible by having gills which open directly into the throat, so that they can continue to respire while still sucking blood. They are sufficiently well adapted in this respect to have survived for over four hundred million years, despite their many other primitive features. But there is one important way in which they have changed: they have not only noses, but also a nose-brain.
The nervous system of these suckers is rudimentary. There are no sympathetic or autonomic nerves at all: none of the networks which, in more modern vertebrates, serve the intestine, the liver, all glands and the heart. But the nerves from the nose gather in a bundle in the head which is already substantial and beginning to spread out sideways, expanding in direct proportion to its employment. The most exciting feature of this growth is that, even in this early stage, it is starting to take on the shape of something new. It is a burgeoning forebrain, bursting into life in direct response to an olfactory need.
In a habitat where sight is limited and sound cannot be localized, only smell can pick up traces that provide solid information from afar. But smell on its own is not enough. There has to be a way of telling where a smell is coming from. Hagfish and lamprey put themselves into an odour corridor by swinging their bodies from side to side, sampling water on a broad front with their single nasal opening. But most later fish have two external 'nares', or nostrils, and practise 'stereo smell', which improves with the distance between the openings. The wider the head, the better the chance of smelling in stereo. And somewhere in the space between the nostrils, there was room for a coordinator: a place where information about smells could be analysed and acted upon.
Fish live in an environment where even substances that are only partially soluble find their way to chemically sensitive areas of the body, making it possible for extraordinary feats of detection. The Common European eel, for instance, has been shown to have a very uncommon affinity for some alcohols, responding to just a few molecules at a time in concentrations as low as one part in [10.sup.18]. This represents the sort of dilution you would get by tipping a single shot of vodka into a body of water the size of Lake Erie. These eels are, of course, teetotallers. But they spawn and die in the depths of the Sargasso Sea, leaving leaflike larvae to make their own way, unguided, across five thousand kilometres of open ocean, back to ancestral haunts in the pools and lakes of Poland and Germany, in a journey that lasts three years. How they do this remains mysterious, but it seems to have something to do with smell.
Experiments with salmon show that they are able to distinguish between plain water and water in which an aquatic plant has been briefly rinsed. Young salmon probably imprint on the particular flavours captured by their home streams from the unique combination of plants that grow only in that watershed. It is difficult to imagine how young eels manage without such crucial early experience, yet they do. They are driven by instinct, and by the presence or absence of just a few special molecules, to make decisions on which the very survival of their species is at stake.
Smell is stimulating. It stirs things up and makes us nostalgic a wonderful word which literally means 'ache for home' which serves to inspire new circuits in the brain. Enough, in simpler species, to form a simple nose-brain. In migratory fish such as salmon, this centre has split into a paired organ, more befitting species that have begun to perceive and to perform bilaterally. These are the olfactory bulbs.
Many sharks find their food by smell. If water in which live fish have been kept is siphoned into a shark tank, the residents respond with hunting behaviour. And if the stream comes from injured or dead fish, they can be provoked into a feeding frenzy. The excitement of the sharks increases directly with signs of stress from their prey. They detect and respond to the smell of trouble, and do so with extraordinary accuracy. In one experiment with a white-tip shark, an injured fish was allowed to swim the length of a tank before taking cover. When the shark was released soon afterwards, it followed exactly along the same zigzag line, duplicating every movement made by the now-invisible prey.
It seems that smell leads these predators to their prey from a distance, but the final move, the kill, may be triggered by another sense. For some species, in clear water and in daylight, this is often vision. In others it may be pressure waves detected by sensory cells along the flank. But in small, bottom-living sharks such as the spotted dogfish, the focus appears to be a weak electrical field produced by another fish's muscles. And all these impressions seem to be enhanced by smell, even linked with it in some strange way.
Such synesthesia, the blending of one sense with another, is vital. Even in the lives of fishes, sensation is seldom a matter of one thing or another. Senses overlap. The lines between them often tend to be blurred, and the best that we can manage, by way of description from the outside, is to say that the senses of fishes appear to dominate one at a time.
In the case of the spotted dogfish, smell slides seamlessly into an awareness which, if the fish could tell us, it might have to describe as a flavour rather than an odour. I find it fascinating that this very dogfish is renowned for having a forebrain which is not only large, but also equipped with a pair of swollen olfactory bulbs, each proportionately larger and better differentiated than those of almost any other fish. It is well prepared for blurring sensory boundaries in the same way that we, when faced with perceptual confusion, resort to metaphors, talking of 'loud smells' or 'bright sounds'.
Dr Johnson experienced the colour scarlet as 'the clangour of a trumpet', and the poet Rimbaud described the sound of the vowel a as 'a black hairy corset of flies'. And it can be no coincidence that natural synesthetes, those who experience intense sensory crossovers on a regular basis, have problems with their limbic systems those areas of the brain that, in mammals, grew out of the old olfactory bulbs.
One physiologist has even described human sensory blenders as 'living cognitive fossils', displaying a memory of how our early vertebrate ancestors once saw, heard, touched, tasted and smelled. It is a fact, too, that our cerebral hemispheres, those walnut-folded swellings of grey matter that dominate the forebrain and now control most of our conscious behaviour, also developed directly from olfactory tissue.
The limits of sensory evolution in fish are defined very largely by their habitat. Water is physically supportive, carries some kinds of odour well, and is kind to sound letting it travel several times faster than air will allow, but it inhibits other more personal kinds of communication. Much more becomes possible when scents are released into the air. Breathing air is a liberating experience. It freed our ancestors from the constraints of staying wet or having to remain within easy reach of water for refuge, respiration or reproduction. But the biggest change it made in our lives was to expose us to a whole new range of sensory experience.
Air is traditionally 'thin', but the more we learn about our atmosphere, the more substantial it becomes. In some places it is so filled with inorganic flotsam that it is almost thick enough to plough; in others, it has become so primed with the by-products of life that it comes close to being a living tissue in its own right. Even the cleanest air, at the centre of the South Pacific or somewhere over Antarctica, has two hundred thousand assorted bits and pieces in every lungful. And this count rises to two million or more in the thick of the Serengeti migration, or over a six-lane highway during rush hour in downtown Los Angeles.
Most airborne material consists of minute particles of salt, clay, and ash from forest fires and distant volcanic eruptions. And mixed in with, or growing on, or simply being carried along by this fertile soil is a garden of exotic flora and fauna. Every lungful of air we borrow from this gruel is likely to contain a few stray viruses in transit between their hosts; four or five common bacteria; fifty or sixty fungi, including several rusts or moulds; one or two minute algae drifting in from the coast; and possibly a fern or moss spore, or even an encysted protozoan.
All of which is inevitable. This is, after all, the stuff of life. We share our planet quite naturally with a permanent aeroplankton; a buoyant ecology too soft to hear, too small to see, but heavy with mood and meaning. Imagine being aware of all these airy inclusions and you can begin to understand how it might feel to be able to smell really well.
Air-breathers do things differently. Their olfactory sense cells lie, not in isolated nasal sacs, as in fishes, but strategically placed in a nasal passage, a thoroughfare through which air passes on its way to the lungs. And that simple fact gives noses a new slant. The difference is most dramatically demonstrated in an intermediate animal, one that has a nose in both worlds, something like a frog or a toad that is amphibious, literally leading a double life. The African clawed toad is the white rat of this arena.
Every gynaecological laboratory has one, largely because it provided the first reliable way of testing for human pregnancy. An unfertilized female toad lays eggs within hours of being injected with urine containing hormones that are characteristic of newly pregnant women. But these smooth, streamlined, strange-footed toads whose triple claws are used for digging for food on the bottom of South African ponds are rapidly becoming equally popular with geneticists.
At the Institute of Zoophysiology in Stuttgart, work on the toad genome has revealed that 'Strange Foot' has an equally strange genetic repertoire. This includes hundreds of genes that code for the way in which its olfactory sense cells (called receptor cells) can function. That is no great surprise: mammals have thousands of such genes while fish have very few, so it was predicted that amphibians would be intermediate between the two. And so they are. Their olfactory genes fall into families of two distinct kinds.
Aquatic animals are exposed to water-soluble molecules such as amino acids, while air-breathers have access to a far greater variety of more volatile odorants. And as an amphibian, adapted to both aquatic and terrestrial life, the clawed toad was expected to have roughly equal numbers of both genes and receptor cells. It doesn't. There are far more cells of the mammalian air-breathing kind, probably because there are far more airborne than water borne odours. But the big surprise is that the two kinds of receptors are found in separate areas. Strange Foot has two noses and two senses of smell: one for use underwater, the other for life in the air.
The toad's nose is neatly divided into two separate sacs or chambers. Just inside each nostril there is a flap of tissue which acts like a valve between them. Underwater, the flap swings to seal off the main chamber, exposing another one, a cul-de-sac filled with receptors sensitive to water-borne odours. And when the toad surfaces and raises its nose into the air, the flap swings the other way to seal off the aquatic chamber, exposing the main one, which is lined with cells sensitive to volatile odours in the air. And after serving this chamber, the airstream passes on to provide oxygen to the lungs.
Living in the air demands more of the sense of smell. But the challenge seems to be one that even the most primitive amphibians are ready to meet.
Mexican toads, in the process of learning to negotiate a maze, have been trained to remember alien scents such as geraniol, vanillin and cedarwood. Both the spotted chorus frog and Strecker's chorus frog have shown that they can pick up the odour of their own breeding ponds many hundreds of metres away. North American leopard frogs, blinded by severing their optic nerves behind the eye, can nevertheless navigate their way back to a familiar pool, whether they are released upwind or downwind of their homes. But it is the olfactory skill of the Californian red-bellied newt that takes one's breath away.
This newt is lizard-like, with large dark eyes and tomato-red markings that warn of a poisonous secretion in its skin. It has few problems with predators in the coastal mountains where it lives, but has been persecuted instead by local biologists interested in its almost unbelievable navigational skills. It can be taken away from its usual damp haunts in one valley, carried across a mountain divide three hundred metres high, and still make its way unerringly back home. If moved in any direction, it will succeed, even in totally strange territory, in orienting itself quickly and directly towards home.
Blinding this nostalgic little animal doesn't slow it down for a moment. And squirting formaldehyde into its nose to kill the epithelium the thin lining of tissue that contains olfactory sense cells results only in what has been described as 'a significant loss of orientation ability'. The only way, it seems, to prevent it from getting home is to sever its olfactory nerves entirely. Such invasive studies distress me. But the fact that they were thought to be necessary is a measure of our frustration when faced with behaviour for which we have no easy explanation. Such mysteries lie in every part of natural history. Even the simplest creatures have the capacity to surprise us when we think boldly enough to ask them the right kinds of questions. And the answers are never easy, except in hindsight.
In the case of the wandering newts, however, there may be a clue. There is something I have so far failed to mention about amphibians, something that began with those frogs and toads whose noses have divided into the two chambers appropriate to their schizophrenic lifestyle. It is this: some of them, including this newt, also have a third nasal space which is not directly involved in detecting smells, either underwater or in the air. And this could be involved in a new and different kind of awareness.
The olfactory sense cells of all vertebrates are, on the whole, surprisingly alike. And also very strange. They are, for a start, unique in that they are in direct contact with the outside world. Most other sense organs lurk under the skin, embedded deep in protective tissue, picking up sensations by remote control. But smell cells go unadorned: naked neurons, each one right out there in the open, like a unicellular organism, meeting molecules, making its own way in the world. And, strangest of all, small cells wear out after a few weeks and need to be replaced on the front lines. They regenerate in ways no other nerve cells in our bodies ever do.
The number of olfactory sensory cells operating at any one time varies from one species to another, depending on the emphasis a species places on having a good sense of smell. Humans, for example, have about six million cells; rabbits fifty million; and sheep dogs over two hundred million.
In all species the cells are thin, with projections, or processes, at either end. One of these extends inside the body, usually through a hole in the skull, to make contact with the brain. The other process is far shorter and stays on the outside in a knob topped with a tassel of fine sensory hairs, called cilia. The number of cilia varies too, according to the species. Domestic cattle, for example, all have exactly twenty-seven microscopic lashes on each sensory cell. Tiny newts have fewer such hairs, but theirs are four times as long.
It is generally agreed that the function of these frills is to bind odour molecules and convert their chemical signals into electrical ones for transmission to the olfactory bulb in the brain. So it is interesting to discover that, in addition to typical sensory cells in the main and side chambers of some amphibian noses, there are other cells in a deeper chamber that look very different. They are flask-shaped, with long necks. They have no cilia, but have knobs decorated with a tuft of short bristles, sometimes called microvilli, that makes them look like sensory cells with a crew-cut. And the tissue in which these bristle cells lie has none of the glands which, in normal olfactory sensory epithelium, produce the mucus that helps to trap and hold airborne chemicals.
No one knows exactly what these strange cells do. I suspect that they give air-breathing animals a new sensory edge. This extra faculty may be related to the sense of smell, and might even partially replace it, but it has a different, more multi-sensory bias, and a whole new perceptual agenda that becomes truly obvious only in the first fully terrestrial vertebrates the reptiles.
Some reptiles differ very little from amphibians in both their lifestyle and their nasal structure. Marine turtles are as water-dependent as clawed toads and have very simple nasal passages that run, almost in a straight line, from the external nostril to an internal opening in the throat. Land tortoises possess larger olfactory structures, and crocodilians have a surprisingly sophisticated series of chambers and sinuses which provide additional surfaces for the usual olfactory sense cells.
Marine snakes, tree-living lizards and chameleons are all conservative about smell, but it is among ground-living lizards and snakes, those closest to the source of many scents, that the greatest changes have taken place. And the most dramatic of these is a concentration of the mysterious bristle cells in a pair of separate chambers of their own. This, finally, is Jacobson's Organ.
The discoverer of the organ, Ludwig Levin Jacobson, was born in 1783 in Copenhagen. He qualified in surgery at the age of just twenty-one and went to study in Paris with the great anatomist Baron Georges Cuvier, who believed that the habits of an animal determine its form. Cuvier also refined the taxonomic system of Linnaeus by showing how all organisms could be classified on the basis of their anatomical differences. And it was probably he who drew Jacobson's attention to the work of Frederick Ruysch, a Dutch embalmer who in 1703 described the anatomy of a number of animals, including a snake with unusual pits in its palate. So Jacobson looked for, in 1809 discovered, and in 1811 published a report on the organ which still bears his name.
The Organ of Jacobson remained a zoological curiosity until the late nineteenth century, when interest in it was revived by one of the most charismatic figures in Victorian science. Robert Broom was a Scot and a physician with a very curious mind. One of the last great individualists, he found fault with a place or a practice only if it offered 'too much medicine and too little natural history'. In later life he became one of the world's great palaeontologists and physical anthropologists, doing pioneer work on the origins of humans in Africa, but his first enthusiasm was for reptiles, their origins and the significance of Jacobson's Organ. In between practising medicine he scoured museum collections and studied animals in the wilds of Australia and South Africa for material to include in a doctoral thesis, 'On the comparative anatomy of Jacobson's Organ', which he submitted to Glasgow University.
'It would seem,' said Broom, 'that the Organ of Jacobson is the organ in the body that is least liable to become altered by change of habit. I can almost identify an animal by examining this organ and often tell of its affinities.' He went on to do just that for the mammal-like reptiles that once roamed South Africa, most of which he found himself and modestly described as 'the most important fossil animals ever discovered' because 'there is little doubt that among them we have the ancestors of mammals and the remote ancestors of human beings'.
Recent research confirms Broom's suspicions about Jacobson's Organ. Signs of it appear, like portents, in the embryos of all higher land mammals, although in some, such as tree-living lizards, aquatic turtles and nearly all birds, it never actually materializes. There is no need for it. In others, such as ground-living lizards and most snakes, the organ comes into being, not simply as pouches off the nasal cavity, but as a pair of blind chambers that open directly into the mouth. Why should this be? What happened to make this development not just useful, but necessary?
Robert Broom, who could put flesh on the bones of a fossil even while it was still in the ground, described minute channels on the skulls of some of his finds in the fossil beds of the Karroo Desert, marks which suggest that Jacobson's Organ arrived on the scene during the Triassic, perhaps two hundred million years ago. It appeared, he believed, to meet a need that could best be understood by looking at lizards or snakes that live today in similar habitats.
The most obvious sensory feature of all living snakes is that they have two olfactory systems. In one, external nostrils lead to nasal cavities containing sensory cells with cilia, embedded in a tissue containing glands. The other consists of cells without cilia, in a tissue without glands or mucus, housed in a pair of dome-shaped structures on either side of the nasal septum. Access to this second system is restricted to a pair of tiny pits in the roof of the mouth, leading to ducts that pass through the bony palate.
The first system is the main olfactory apparatus, often simply known as the organ of smell, though neither of those descriptions is totally accurate. It is not the only organ, nor even necessarily the main one, involved in smell. The second is Jacobson's Organ, comprising a rival chemical sense system that is becoming the focus of very active research and debate, much of it based on one common little snake that has been studied in the field by David Crews and his colleagues at the University of Texas.
Garter snakes are the most widely distributed reptiles in North America. They can be found at all altitudes, ranging from coast to coast and from Canada all the way south to Costa Rica. They are slender, graceful snakes, seldom more than sixty centimetres long, marked with dark spots and longitudinal red stripes, giving them some resemblance to those fancy coloured garters that once supported every gentleman's socks. But their appeal to biologists in the last two decades is more sensory than sartorial.
Plains garter snakes in cool areas have the habit of gathering, ten thousand at a time, in dens established in underground caverns where they spend the long winters in hibernation. At these times their blood becomes as thick as mayonnaise, and they barely move for up to six months. But in May, as soon as the outside temperature reaches 25°C, dormancy ends and the tangle of bodies stirs and turns into a writhing mass that flows out of the den.
First the males emerge en masse and wait near the entrance, sunning themselves, showing no interest in food or drink. Then the slightly larger females come out, one at a time, to find themselves heavily outnumbered and soon entangled in a mating ball of perhaps a hundred hopeful suitors. Fifteen minutes later, carrying a load of sperm in her oviducts, each solitary female sets off on a migration to summer feeding grounds, where she will restore her body fats and give birth to up to thirty live young garters in the autumn. And by late September, she and thousands of her kind gather once again in the familiar scent of some shelter or hibernaculum.
This busy cycle of mating, migration, feeding, gestating and returning to hibernation, spans little more than the three summer months and presents a number of zoological problems. Some are easily resolved. The sperm each female receives in spring is stored in her oviduct for up to eight weeks, until her follicles are ready to be fertilized in midsummer. The male's reproductive organs also build up post-nuptially, expanding rapidly during the summer, when the living is easy, and he goes into hibernation packed with sperm, ready for the following spring, when the population is concentrated once more and mating is most likely to succeed. Other questions raised by the behaviour of garter snakes took longer to answer.
(Continues...)
Table of Contents
Right under our noses | ix | |
Part 1 | Getting Organized | 1 |
Fragrantes | 11 | |
1 | A nose is born | 12 |
Hircinos | 34 | |
2 | In warm blood | 35 |
Ambrosiacos | 55 | |
3 | On the net | 56 |
Part 2 | The Fragrant Ape | 77 |
Tetros | 84 | |
4 | Scents of self | 85 |
Nauseosos | 112 | |
5 | In bad odour | 114 |
Aromaticos | 141 | |
6 | Smelling good | 142 |
Part 3 | The Most Human Thing | 175 |
Alliaceos | 184 | |
7 | The sixth sense | 186 |
Taxonomy | 217 | |
A Note on the Illustrations | 221 | |
Bibliography | 222 | |
Index | 237 |