Modern Styrenic Polymers: Polystyrenes and Styrenic Copolymers / Edition 1 available in Hardcover
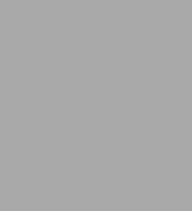
Modern Styrenic Polymers: Polystyrenes and Styrenic Copolymers / Edition 1
- ISBN-10:
- 0471497525
- ISBN-13:
- 9780471497523
- Pub. Date:
- 03/28/2003
- Publisher:
- Wiley
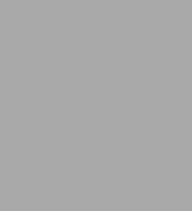
Modern Styrenic Polymers: Polystyrenes and Styrenic Copolymers / Edition 1
Hardcover
Buy New
$718.95-
SHIP THIS ITEMIn stock. Ships in 1-2 days.PICK UP IN STORE
Your local store may have stock of this item.
Available within 2 business hours
Overview
- It covers the advent of branched polystyrenes, syndiotactic polystyrene, high-molecular weight general purpose PS, styrenic interpolymers, and clear SBS copolymers
- Presents voluminous research previously only reported at conferences in one reference
- Unique coverage of a topic not found in the field
Product Details
ISBN-13: | 9780471497523 |
---|---|
Publisher: | Wiley |
Publication date: | 03/28/2003 |
Series: | Wiley Series in Polymer Science , #6 |
Pages: | 792 |
Product dimensions: | 6.30(w) x 9.33(h) x 2.06(d) |
About the Author
Duane Priddy is the editor of Modern Styrenic Polymers: Polystyrenes and Styrenic Copolymers, published by Wiley.
Read an Excerpt
Modern Styrenic Polymers
Polystyrenes and Styrenic CopolymersJohn Wiley & Sons
Copyright © 2003 John Wiley & Sons LtdAll right reserved.
ISBN: 0-471-49752-5
Chapter One
Historical Overview of Styrenic PolymersJOHN SCHEIRS ExcelPlas, Edithvale, VIC, Australia
1 INTRODUCTION
Styrene has been known from the mid-nineteenth century as a clear organic liquid of characteristic pungent sweet odour. It was also known to have the ability to convert itself under certain conditions into a clear resinous solid that is almost odour-free, this resin then being referred to as 'metastyrol'.
Styrene readily polymerizes in air and it is therefore not surprising that there are a number of early obscure reports referring to its 'polymerization' predating 1900. However, because the concept of polymerization had not yet been proposed (until Staudinger in 1920), many of these early reports referred to the 'oxidation' or 'hardening' of the styrene monomer.
In 1839, Eduard Simon, an apothecary in Berlin, distilled storax resin obtained from the 'Tree of Turkey', (liquid ambar orientalis) with a sodium carbonate solution and obtained an oil which he analysed and named styrol (what we now call styrene). He recorded the following observation: 'that with old oil the residue which cannot be vaporised without decomposition is greater than with fresh oil, undoubtedly due to a steady conversion of the oil by air, lightand heat to a rubberlike substance'. Simon believed he had oxidised the material and called the product styrol oxide. Later, when he realised that it contained no oxygen, the product became known as metastyrol. This puzzled the early chemists as there was no change in empirical formula despite the very pronounced alteration in chemical and physical properties. Unknowingly, this was the first recorded instance of polymerization.
A few years later, in 1845, Blyth and Hofmann observed that 'metastyrol' was formed when styrene was exposed to sunlight, while it remained unchanged in the dark. This is the first report of photopolymerization.
It was established by Blyth and Hofmann in 1845 that 'styrol' and its conversion product 'metastyrol' had the same elemental composition with an equal number of carbon and hydrogen atoms. After nitration, the N:C ratio was 1:8 for styrol and 1:7 for metastyrol, leading to the conclusion that [C.sub.8][H.sub.8] had been converted to [C.sub.7][H.sub.7]. However, the conclusion that styrol had polymerized was not reached until 75 years later.
The first samples of polystyrene were characterized by the German organic chemist Staudinger. It was observed that the polystyrene could be fractionated into samples with different solution viscosities and this observation was incompatible with the notion that the substance was a colloidal aggregate. Staudinger challenged the notion that polymeric substances are held together by 'association forces'. It was Staudinger who first realised that the solid that Simon had isolated from natural resin was in fact composed of long chains of styrene molecules. Staudinger postulated that polystyrene was a high molecular weight polymer. His critics argued that it could not be a high molecular weight polymer because of its solubility in common solvents. He introduced the term 'macromolecules' to describe these long-chain compounds. Fierce controversy with his colleagues caused Staudinger to move from the Swiss Federal Institute of Technology in Zurich (ETH) to the University of Freiburg.
In 1929, Staudinger and co-workers also synthesized hexahydropolystyrene by the nickel-catalysed hydrogenation of polystyrene. The hydrogenated polystyrene, also known as poly(cyclohexylethylene), had improved oxidative and radiation stability relative to conventional polystyrene.
It was also Staudinger in 1932 who first proposed that the inability of polystyrene to crystallize was due to its lack of stereoregularity which rendered it amorphous. It is its amorphous nature that is responsible for its solubility - though others claimed that polymer solubility was incompatible with very high molecular weight.
2 GENERAL-PURPOSE POLYSTYRENE (GPPS)
Styrene readily polymerizes to polystyrene (PS) either thermally or with free-radical initiators. A limiting factor in the commercial exploitation of polystyrene was the high reactivity and considerable heat of polymerization of styrene. The polymerization rate of styrene is exceedingly fast and considerable heat is generated. This was an intimidating obstacle to commercial production of PS since many in the industry were concerned that the large-scale polymerization of styrene may result in a dangerous uncontrolled reaction. The process involved heating styrene monomer in bulk containers. A major limitation of this approach was the need for heat removal from the highly viscous melt. High temperatures can be reached in large mass reactors (>300°C) and thermal degradation of the resultant PS can occur. This problem was later solved by installing heat exchanger tubes in the reaction medium. The first commercial production of PS was in 1931 by BASF.
To prevent premature polymerization of styrene monomer, special inhibitors had to be added so that it could be stored until needed. Polymerization inhibitors were also required to prevent polymer formation during distillation of styrene monomer from ethylbenzene.
Before the large-scale production of PS could occur, a consistent supply of styrene monomer was required. In 1930, Dow started to produce styrene monomer by cracking its ethylbenezene precursor. In 1938, Dow began manufacturing commercial quantities of PS.
The propensity for styrene monomer to polymerize allowed extremely simple and crude polymerization techniques. The first technique used by Dow was known as the 'can process' since it basically involved filling 10 gallon metal cans (Figure 1.1) with styrene monomer followed by heating the cans in a heating bath at progressively higher temperatures for a number of days. After this time the polystyrene (polymerized to approximately 99% conversion) was removed from the can and crushed to a free-flowing powder.
The development of styrene and PS manufacturing technology was spurred on by the advent of World War II. During this time the supply of natural rubber from the Far East was terminated. The acute rubber shortage accelerated the development of styrene-based synthetic rubber. With the outbreak of the war, the United States embarked on a scientific programme that rivalled the Manhattan Project in its scope and significance. Nearly a billion dollars was spent on research and development of synthetic rubber needed to keep the Allied war effort in motion. The key players such as Dow, Monsanto and Koppers Chemical cooperatively produced record quantities of styrene monomer for the preparation of styrene-butadiene rubber. A staggering 180 000 t of styrene monomer were produced per year towards the end of the War with most being used for the production of the synthetic rubber Buna-S (also known as GRS rubber, where GR stands for government rubber and S for styrene). There was also cooperation between the main rubber-producing companies, Goodyear, B. F. Goodrich, Standard Oil, Firestone and US Rubber.
In early 1942, the American Synthetic Rubber Research Program commenced. Along with the major rubber-producing companies, 11 university research groups, including Carl 'Speed' Marvel at the University of Illinois, Izaak 'Piet' Kolthoff at the University of Minnesota and W. D. Harkins and Morris Kharasch of the University of Chicago, joined the effort to make synthetic rubber work. Their objective was to set up four plants that would produce 30 000 t each of synthetic rubber per year. By the end of 1942, four plants were established but their output was under the target. By the end of 1943, 15 plants were in operation, and supply had begun to meet demand.
The research focus during the War was on refinement, enhancement and incremental improvement of existing processes. For example, if the rubber is allowed to polymerize until no monomer is left then long, branched molecules are produced, which gel and make the rubber difficult to process. To solve this problem, the reaction is only allowed to proceed to 72% conversion and a thiol modifier, a chain-transfer agent, was used to control molecular weight. It was also observed that the polymerizations have an 'induction period' which varied from batch to batch. During the induction period nothing seems to be happening, then, all of the sudden, the reaction takes off. The researchers at the University of Illinois found that this is due to different fatty acids present in the different soaps needed for the emulsion process. These soaps also cause the solution to foam during the recovery of the remaining monomer. This problem leads to the development of silicone defoamers.
The properties of the Buna-S type rubber are highly dependent on the amount of styrene in the rubber. To determine properties, it is important to know how much styrene had been incorporated. William O. Baker of Bell Telephone Laboratories solved this problem by developing a procedure for determining the amount of styrene using the refractive index of a solution of the rubber.
It was not until after World War II, when styrene monomer capacity could be diverted from its essential wartime use for styrene-butadiene synthetic rubber, that polystyrene became an important commercial plastic. When the War finished the supply route for natural rubber was re-established and there was an oversupply of styrene monomer. The extensive infrastructure for styrene production and the enormous body of process and technical knowledge laid the foundation for the post-War development of polystyrene and styrenic copolymers.
Prior to 1941, Germany had a major technical and industrial lead over the USA, having already established an industrial styrene monomer production process, a styrene-butadiene elastomer process and a mass styrene polymerization process. Figure 1.2 shows the polymerization vessels at I. G. Farben in 1940. Figure 1.3 shows a bank of polymerization kettles. The Germans began the first technical production of polystyrene in 1930 while the first production of polystyrene in the USA was some 8 years later by Dow in 1938.
Interestingly, at the inception Dow did not have a strategic objective to enter into the polystyrene business. Rather, Dow believed that ethylcellulose and poly (vinylene chloride) (Saran) were the commodity polymers of the future. Dow was producing ethylbenzene as a solvent and electrical fluid. However, when the markets for ethylbenzene did not develop it decided to crack the ethylbenzene and produce styrene. After it had stockpiled large quantities of unstable styrene, Dow initiated a 'crash' programme to develop polystyrene. Thus even though Dow did not initially intend to produce polystyrene commercially when its petrochemical programme was initiated it became a logical business decision to do so.
Early on there were numerous technical barriers that made polystyrene difficult to produce and to process. For example, it was made by an extremely slow production process and its high average molecular weight and broad molecular weight distribution made it difficult to injection mould. Dow researchers ultimately developed ways to lower the average molecular weight and added certain lubricants to improve processability, thus making general-purpose polystyrene which fast acquired the reputation of being the easiest thermoplastic to mould.
Other technical barriers were the need to control the exotherm of polymerization and to produce colour-free polystyrene. While the manufacture of styrene seems simple and straightforward, in the early days at Dow there were three major impurities in the styrene monomer apart from residual ethyl-benzene. These were phenylacetylene (which acted as an inhibitor for styrene polymerization), divinylbenzene (which caused plugging and fouling of the distillation column for separating styrene from its precursor, ethyl benzene) and sulphur (which caused discoloration of the polystyrene).
Finally, in 1938, the 'crash' programme resulted in the first saleable polystyrene batches. This was produced in metal cans lined with tin to yield high-purity polystyrene. These cans were filled with styrene and immersed in heated water-baths where the styrene would thermally polymerize. The process was very slow and labour intensive. Further, the exotherm of polymerization was greatest in the centre of each can, which led to a core of lower than average molecular weight. After polymerization was complete, the polystyrene billet was ground up and mixed to distribute the different molecular weight regions.
Although the can process was very slow, it lent itself to easy expansion of production output by simply adding more cans and heating baths. It was also discovered that by adding some peroxide catalyst to the styrene monomer the production throughput could be increased significantly. In fact, this innovation led to a doubling of Dow's plant capacity since faster polymerization rates could be achieved while still controlling the exotherm.
While the USA was progressing with the can process, Germany had already developed a continuous process for the mass polymerization of styrene.
After World War II, researchers from Dow visited the German polystyrene plants and were surprised to learn of their scale and sophistication. One of the key people on the investigating team that went to Germany was Dr Goggin, founder of Dow's Plastics Technical Service Department. The American teams that visited I. G. Farben after the War recorded their findings in a historic report. This report clearly showed the advantages of a continuous production process for polystyrene. Further, the first industrial production of SAN was in 1936 also by I. G. Farben in Ludwigshafen.
Central to Germany's development of polystyrene technology was Herman F. Mark (Figure 1.4). Mark worked at I. G. Farben Industrie for 6 years from 1927 to 1932, first as a research chemist (1927-28), then as Group Leader (1928-30) and finally as Assistant Research Director (1930-32). Because of the changing political climate, Mark moved to the University of Vienna, where he became Professor of Chemistry and Director of the First Chemical Institute (1932-38). While at I. G. Farben Industrie, Mark played a major role in the development of styrene monomer and PS. Mark patented a process in 1929 for the production of styrene from ethylbenzene via catalytic dehydrogenation.
The German Chemical giant I. G. Farben developed the continuous tower process for PS in the 1930s. The German PS polymerization plant shown in Figure 1.5 overcame the problem of the polymerization exotherm and thermal runaway by using a tank reactor with heat-transfer tubes criss-crossed through it.
Continues...
Excerpted from Modern Styrenic Polymers Copyright © 2003 by John Wiley & Sons Ltd. Excerpted by permission.
All rights reserved. No part of this excerpt may be reproduced or reprinted without permission in writing from the publisher.
Excerpts are provided by Dial-A-Book Inc. solely for the personal use of visitors to this web site.
Table of Contents
Contributors.Series Preface.
Preface.
About the Editors.
I: INTRODUCTION TO STYRENIC POLYMERS.
Historical Overview of Styrenic Polymers (J. Scheirs).
Polystyrene and Styrene Copolymers - An Overview (N. Niessner and H. Gausepohl)
II: PREPARATION OF STYRENIC POLYMERS.
Commercial Processes for the Manufacture of Polystyrene (B. J. Meister and C. J. Cummings)
Approaches to Low Residual Polystyrene (D. B. Priddy).
Process Modelling and Optimization of Styrene Polymerization (J. Gao, K. D. Hungenberg and A. Penlidis).
Living Free Radical Polymerization of Styrene (A. Butté, G. Storti and M. Morbidelli).
Increasing Production Rates of High MW Polystyrene (B. Matthews and D. B. Priddy).
Preparation of Styrene Block Copolymers Using Nitroxide Mediated Polymerization (D. B. Priddy.).
III: MAJOR CLASSES OF STYRENIC POLYMERS.
Particle Foam Based on Expandable Polystyrene (EPS) (R.-D. Klodt and B. Gougeon).
Rigid Polystyrene Foams and Alternative Blowing Agents (K. W. Suh and A. N. Paquet).
Polystyrene Packaging Applications: Foam Sheet and Oriented Sheet (G. C. Welsh).
Preparation, Properties and Applications of High-impact Polystyrene (M. F. Martin, J. P. Viola and J. R. Wuensch).
Key Structural Features Impacting SAN Copolymer Performance (R. P. Dion and R. L. Sammler).
Rubber Particle Formation in Mass ABS (G. Bouquet).
High Heat Resistant ABS Technology (R. Vanspeybroeck, R. P. Dion and J. M. Ceraso).
Synthesis, Properties and Applications of Acrylonitrile-Styrene-Acrylate Polymers (G. E. McKee, A. Kistenmacher, H. Goerrissen and M. Breulmann).
IV: SYNDIOTACTIC POLYSTYRENE.
Synthesis of Syndiotactic Polystyrene (N. Tomotsu, M. Malanga and J. Schellenberg).
Characterization, Properties and Applications of Syndiotactic Polystyrene (K. Yamasaki, N. Tomotsu and M. Malanga).
Rubber Modification of Syndiotactic Polystyrene (G. E. McKee, F. Ramsteiner and W. Heckmann).
Polymeric Blends Based on Syndiotactic Polystyrene (L. Abis, R. Braglia, G. Giannotta and R. Pò).
V: STYRENIC BLOCK COPOLYMERS.
Styrenic Block Copolymer Elastomers (R. C. Bening, W. H. Korcz and D. L. Handlin, Jr).
Preparation, Properties and Applications of High Styrene Content Styrene-Butadiene Copolymers (D. L. Hartsock and N. E. Stacy).
VI: NOVEL POLYSTYRENES.
Hydrogenated Polystyrene: Preparation and Properties (S. F. Hahn).
Branched Polystyrene (K. Koppi and D. B. Priddy).
'Super Polystyrene' - Styrene-Diphenylethylene Copolymers (G. E. McKee, F. Ramsteiner, W. Heckmann and H. Gausepohl).
Ethylene-Styrene Copolymers (Y. W. Cheung and M. J. Guest).
VII: PROPERTIES OF STYRENIC POLYMERS.
Fracture Behaviour of High-impact Polystyrene and Acrylonitrile-Butadiene-Styrene (T. Vu-Khanh).
Dynamic Mechanical Behaviour of Atactic Polystyrene, High-impact Polystyrene and Other Styrenic Polymers (S. N. Goyanes and G. H. Rubiolo).
Flame-retardant Polystyrene: Theory and Practice (B. King).
Photochemical Degradation of Styrenic Polymers (B. Mailhot, A. Rivaton and J. L. Gardette).
Analysis and Levels of Styrene Dimers and Trimers in Polystyrene Food Containers (H. Sakamoto).
Index.
What People are Saying About This
“...useful for practicing polymer scientists and engineers who desire a wide-ranging resource on polystyrene materials...the brad range of subjects addressed support its use as a textbook for studying the general field of polymer science and engineering through the examination of a single material.” (Journal of the American Chemical Society, Volume 126, 4741)
"...a wonderful book...this is a 'breath taking' accomplishment having great value to those involved with styrenics or having interest in styrenics..." (Polymer News)