Molecular Biology of the Cell / Edition 6 available in Hardcover
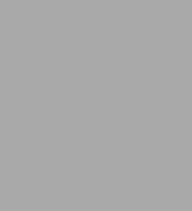
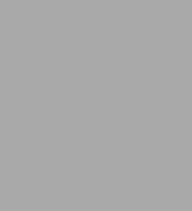
Buy New
$223.75Buy Used
$174.52-
SHIP THIS ITEM— This Item is Not Available
-
PICK UP IN STORECheck Availability at Nearby Stores
Available within 2 business hours
This Item is Not Available
-
SHIP THIS ITEM
Temporarily Out of Stock Online
Please check back later for updated availability.
This Item is Not Available
Overview
The hallmark features of previous editions continue in the Fourth Edition. The book is designed with a clean and open, single-column layout. The art program maintains a completely consistent format and style, and includes over 1,600 photographs, electron micrographs, and original drawings by the authors. Clear and concise concept headings introduce each section. Every chapter contains extensive references. Most important, every chapter has been subjected to a rigorous, collaborative revision process where, in addition to incorporating comments from expert reviewers, each co-author reads and reviews the other authors' prose. The result is a truly integrated work with a single authorial voice.
Product Details
ISBN-13: | 2900815344321 |
---|---|
Publication date: | 11/18/2014 |
Pages: | 1464 |
Product dimensions: | 6.50(w) x 1.50(h) x 9.50(d) |
About the Author
Read an Excerpt
Chapter 1: The Evolution of the Cell
All living creatures are made of cells-small membrane-bounded compartments filled with a concentrated aqueous solution of chemicals. The simplest forms of life are solitary cells that propagate by dividing in two. Higher organisms, such as ourselves, are like cellular cities in which groups of cells perform specialized functions and are linked by intricate systems of communication. Cells occupy a halfway point in the scale of biological complexity. We study them to learn, on the one hand, how they are made from molecules and, on the other, how they cooperate to make an organism as complex as a human being.All organisms, and all of the cells that constitute them, are believed to have descended from a common ancestor cell through evolution by natural selection. This involves two essential processes: (1) the occurrence of random variation in the genetic information passed from an individual to its descendants and (2) selection in favor of genetic information that helps its possessors to survive and propagate. Evolution is the central principle of biology, helping us to make sense of the bewildering variety in the living world.
This chapter, like the book as a whole, is concerned with the progression from molecules to multicellular organisms. It discusses the evolution of the cell, first as a living unit constructed from smaller parts and then as a building block for larger structures. Through evolution, we introduce the cell components and activities that are to be treated in detail, in broadly similar sequence, in the chapters that follow. Beginning with the origins of the first cell on earth, we consider how the properties of certaintypes of large molecules allow hereditary information to be transmitted and expressed and permit evolution to occur. Enclosed in a membrane, these molecules provide the essentials of a self-replicating cell. Following this, we describe the major transition that occurred in the course of evolution, from small bacteriumlike cells to much larger and more complex cells such as are found in present-day plants and animals. Lastly, we suggest ways in which single free-living cells might have given rise to large multicellular organisms, becoming specialized and cooperating in the formation of such intricate organs as the brain.
Clearly, there are dangers in introducing the cell through its evolution: the large gaps in our knowledge can be filled only by speculations that are liable to be wrong in many details. We cannot go back in time to witness the unique molecular events that took place billions of years ago. But those ancient events have left many traces for us to analyze. Ancestral plants, animals, and even bacteria are preserved as fossils. Even more important, every modern organism provides evidence of the character of living organisms in the past. Present-day biological molecules, in particular, are a rich source of information about the course of evolution, revealing fundamental similarities between the most disparate of living organisms and allowing us to map out the differences between them on an objective universal scale. These molecular similarities and differences present us with a problem like that which confronts the literary scholar who seeks to establish the original text of an ancient author by comparing a mass of variant manuscripts that have been corrupted through repeated copying and editing. The task is hard, and the evidence is incomplete, but it is possible at least to make intelligent guesses about the major stages in the evolution of living cells.
From Molecules to the First Cell 1
Simple Biological Molecules Can Form Under Prebiotic Conditions 1, 1 The conditions that existed on the earth in its first billion years are still a matter of dispute. Was the surface initially molten? Did the atmosphere contain ammonia, or methane? Everyone seems to agree, however, that the earth was a violent place with volcanic eruptions, lightning, and torrential rains. There was little if any free oxygen and no layer of ozone to absorb the ultraviolet radiation from the sun. The radiation, by its photochemical action, may have helped to keep the atmosphere rich in reactive molecules and far from chemical equilibrium. Simple organic molecules (that is, molecules containing carbon) are likely to have been produced under such conditions. The best evidence for this comes from laboratory experiments. If mixtures of gases such as COZ, CH4, NH3, and HZ are heated with water and energized by electrical discharge or by ultraviolet radiation, they react to form small organic molecules-usually a rather small selection, each made in large amounts (Figure 1-1). Among these products are compounds, such as hydrogen cyanide (HCN) and formaldehyde (HCHO), that readily undergo further reactions in aqueous solution (Figure 1-2). Most important, representatives of most of the major classes of small organic molecules found in cells are generated, including amino acids, sugars, and the purines and pyrimidines required to make nucleotides.
Although such experiments cannot reproduce the early conditions on the earth exactly, they make it plain that the formation of organic molecules is surprisingly easy. And the developing earth had immense advantages over any human experimenter; it was very large and could produce a wide spectrum of conditions. But above all, it had much more time-tens to hundreds of millions of years. In such circumstances it seems very likely that, at some time and place, many of the simple organic molecules found in present-day cells accumulated in high concentrations.
Complex Chemical Systems Can Develop in an Environment That Is Far from Chemical Equilibrium Simple organic molecules such as amino acids and nucleotides can associate to form polymers. One amino acid can join with another by forming a peptide bond, and two nucleotides can join together by a phosphodiester bond. The repetition of these reactions leads to linear polymers known as polypeptides and polynucleotides, respectively. In present-day living cells, large polyp eptides-known as proteins-and polynucleotides-in the form of both ribonucleic acids (RNA) and deoxyribonucleic acids (DNA)-are commonly viewed as the most important constituents. A restricted set of 20 amino acids constitute the universal building blocks of the proteins, while RNA and DNA molecules are constructed from just four types of nucleotides each. Although it is uncertain why these particular sets of monomers were selected for biosynthesis in preference to others that are chemically similar, we shall see that the chemical properties of the corresponding polymers suit them especially well for their specific roles in the cell.
The earliest polymers may have formed in any of several ways-for example, by the heating of dry organic compounds or by the catalytic activity of high concentrations of inorganic polyphosphates or other crude mineral catalysts. Under laboratory conditions the products of similar reactions are polymers of variable length and random sequence in which the particular amino acid or nucleotide added at any point depends mainly on chance (Figure 1-3). Once a polymer has formed, however, it can itself influence subsequent chemical reactions by acting as a catalyst.
The origin of life requires that in an assortment of such molecules there must have been some possessing, if only to a small extent, a crucial property: the ability to catalyze reactions that lead, directly or indirectly, to production of more molecules of the catalyst itself. Production of catalysts with this special self-promoting property would be favored, and the molecules most efficient in aiding their own production would divert raw materials from the production of other substances. In this way one can envisage the gradual development of an increasingly complex chemical system of organic monomers and polymers that function together to generate more molecules of the same types, fueled by a supply of simple raw materials in the environment. Such an autocatalytic system would have some of the properties we think of as characteristic of living matter: it would comprise a far from random selection of interacting molecules; it would tend to reproduce itself; it would compete with other systems dependent on the same feedstocks; and if deprived of its feedstocks or maintained at a wrong temperature that upsets the balance of reaction rates, it would decay toward chemical equilibrium and "die." But what molecules could have had such autocatalytic properties? In presentday living cells the most versatile catalysts are polypeptides, composed of many diff,prent amino acids with chemically diverse side chains and, consequently, able to adopt diverse three-dimensional forms that bristle with reactive sites. But although polypeptides are versatile as catalysts, there is no known way in which one such molecule can reproduce itself by directly specifying the formation of another of precisely the same sequence.
Polynucleotides Are Capable of Directing Their Own Synthesis 3
Polynucleotides have properties that contrast with those of polypeptides. They have more limited capabilities as catalysts, but they can directly guide the formation of exact copies of their own sequence...
Table of Contents
- Special Features ..... xv
- List of Topics ..... xvii
- Acknowledgments..... xxxix
- A Note to the Reader ..... xliii
- List of Topics ..... xvii
- Part I: Introduction to the Cell ..... 3
- Chapter 1: The Evolution of the Cell ..... 3
- Chapter 2: Small Molecules, Energy, and Biosynthesis ..... 41
- Chapter 3: Macromolecules: Structure, Shape, and Information ..... 89
- Chapter 4: How Cells Are Studied ..... 139
- Chapter 2: Small Molecules, Energy, and Biosynthesis ..... 41
- Part II: Molecular Genetics ..... 195
- Chapter 5: Protein Function ..... 195
- Chapter 6: Basic Genetic Mechanisms ..... 223
- Chapter 7: Recombinant DNA Technology ..... 291
- Chapter 8: The Cell Nucleus ..... 335
- Chapter 9: Control of Gene Expression ..... 401
- Chapter 6: Basic Genetic Mechanisms ..... 223
- Part III: Internal Organization of the Cell ..... 477
- Chapter 10: Membrane Structure ..... 477
- Chapter 11: Membrane Transport of Small Molecules and the Ionic Basis of Membrane Excitability ..... 507
- Chapter 12: Intracellular Compartments and Protein Sorting ..... 551
- Chapter 13: Vesicular Traffic in the Secretory and Endocytic Pathways ..... 599
- Chapter 14: Energy Conversion: Mitochondria and Chloroplasts ..... 653
- Chapter 15: Cell Signaling ..... 721
- Chapter 16: The Cytoskeleton ..... 787
- Chapter 17: The Cell-Division Cycle ..... 863
- Chapter 18: The Mechanics of Cell Division ..... 911
- Chapter 11: Membrane Transport of Small Molecules and the Ionic Basis of Membrane Excitability ..... 507
- Part IV: Cells in Their Social Context ..... 949
- Chapter 19: Cell Junctions, Cell Adhesion, and the Extracellular Matrix ..... 949
- Chapter 20: Germ Cells and Fertilization ..... 1011
- Chapter 21: Cellular Mechanisms of Development ..... 1037
- Chapter 22: Differentiated Cells and the Maintenance of Tissues ..... 1139
- Chapter 23: The Immune System ..... 1195
- Chapter 24: Cancer ..... 1255
- Glossary ..... G-1
- Index ..... I-1
- Chapter 20: Germ Cells and Fertilization ..... 1011