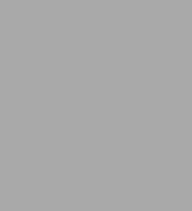
Systems Engineering with Economics, Probability and Statistics: Second Edition
624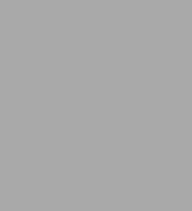
Systems Engineering with Economics, Probability and Statistics: Second Edition
624Hardcover(New Edition)
-
SHIP THIS ITEMIn stock. Ships in 6-10 days.PICK UP IN STORE
Your local store may have stock of this item.
Available within 2 business hours
Related collections and offers
Overview
Product Details
ISBN-13: | 9781604270556 |
---|---|
Publisher: | Ross, J. Publishing, Incorporated |
Publication date: | 01/01/2012 |
Edition description: | New Edition |
Pages: | 624 |
Product dimensions: | 7.50(w) x 9.25(h) x 1.50(d) |
About the Author
Read an Excerpt
CHAPTER 1
Mapping the Terrain of the Systems Approach
1.1 INTRODUCTION
In essence, this book is about using the systems approach to make decisions. It answers the basic question: How can we choose the best course of action, taking into account the goals we are trying to achieve and the constraints that limit our action, by such factors as time, labor, money, and the policies set by the government or by a private organization? Our main purpose is to give the widest possible overview of systems engineering to a beginning engineering student and to explain how a combination of the principles of probability and statistics, economics, and systems analysis can be used for solving engineering problems related to planning, design, and management.
This chapter maps out the terrain of what is covered in succeeding chapters and also describes some preliminary definitions connected with science and systems engineering. How would an engineer minimize the capital and maintenance cost of a long-span bridge? How could an engineer advise his client how to maximize (or optimize) the total income from a high-rise building? What would be the best way to maximize the safety of the railroad system running through your city? Should the government subsidize persons buying an electric car to boost the economy of scale of electric car manufacturers? Should the city extend the light-rail system in San Diego and what would be the implications and consequences attached to this decision? These are the kinds of questions you, as an engineer, planner, or manager, will have to face when you take up a responsible position with a public or private undertaking. To tackle such questions you will need a basic knowledge of economics (both capital budgeting and microeconomics), the principles of probability and statistics, and a working knowledge of systems engineering. All of these topics are covered in this book.
A professional engineer must understand and apply the basic laws of mathematics, physics, chemistry, and economics for planning, designing, managing, and operationalizing engineering works. With hundreds of different recognized engineering specialties, a simple yet comprehensive definition of engineering is:
Engineering is the profession in which knowledge of the mathematical and physical sciences gained by study, experience, and practice is applied with judgment to develop ways to utilize economically, the materials and forces of nature for the progressive well being of society (Crandall and Seabloom, 1970).
It is a concern related to economics that distinguishes engineering from pure science. While economic considerations may be of little or no concern to the pure scientist, the function of the engineer is to utilize the principles of economics to achieve a more efficient and economical end product, such as highways, buildings, water-supply systems, and so on. And it is the evolution of this end product from its conception to its final production, using the creative processes, that is known as engineering design. Design is both an art and a science in that it is a creative problem solving process in which the engineer works within the bounds of a limited monetary budget, a prescribed time line, and specific laws and regulations to convert data, information, technical know-how, combined with his/her ideas, into an accepted product. When an engineering design is finally approved by those authorized to do so, the finished design can then be implemented (Crandall and Seabloom, 1970).
1.2 THE NATURE OF SCIENCE
All engineers invariably take several courses in mathematics and science because these courses form the backbone of engineering science. In a broad sense, science is a way of acquiring testable knowledge about the world. It is now recognized that the knowledge we gain from the scientific approach is provisional and probabilistic, because it is possible that additional experiments carried out by scientists may alter what we already know. Naturally all theories and laws that we currently know are really approximations of the truth within a certain domain of validity.
Some important characteristics of the scientific approach are:
Hypothesis setting and testing: Scientists make propositions or suppositions for reasoning, investigation, or experimentation, for a limited number of variables. Experiments are then conducted to test the hypothesis, holding all other variables constant. If the hypothesis turns out to be correct, it adds to our current knowledge base. If not, the results are rejected.
Replicability: Scientific knowledge must be as objective as possible, which means that a number of observers performing the same experiment, independent of one another but under the same conditions and assumption, should be able to replicate results and verify the observations. This is the scientists' way of verifying (or validating) or falsifying (or rejecting) a proposed hypothesis.
Refutability: While it is impossible for scientists to conduct all possible experiments on a particular topic, due to time constraints, it is important to perform valid experiments using appropriate scientific techniques to decide between competing hypotheses. Although many scientists tend to have their theories corroborated by effective scientific techniques, it is quite possible that these theories could be refuted through a series of additional experiments.
Reductionism. The real world under study is so complex and messy that scientists can only perform simple experiments to capture and comprehend it. As a result, scientists experiment with small units or entities of the real world that can explain cause and effect in a linear way. This style of thinking and experimentation, called reductionism, isolates the phenomenon under investigation from its environment, which eventually produces a mechanistic view of the world (Checkland, 1999; Flood and Carson, 1993).
According to the scientific method, all genuine inquiry and knowledge should be based on hard facts, experimentation, and explanation. It goes further in believing that the methods of science are applicable to all enquiry, especially that of the human and social sciences. This traditional scientific approach has been debated and attacked by many scientists and philosophers, and we take up this debate while considering soft systems thinking in Chapter 12.
1.3 ENGINEERING PLANNING, DESIGN, AND MANAGEMENT
The planning and designing of a product are basic tasks undertaken by engineers to produce an end product. Planning is the arrangement of specific steps for the attainment of an objective. It is a future-oriented and prescriptive process because it assumes our ability to control our own destiny, at least within certain limits. In the context of engineering, planning generally involves the arrangement of spatial patterns over time. However, it must be remembered that it is not the spatial patterns that are planning: they are just the objects of a process. Management, on the other hand, is the skillful use of means (e.g., technology) to accomplish certain ends (e.g., objectives). Engineering design, defined by the Accreditation Board for Engineering and Technology (ABET), is: The process of devising a system, component, or process to meet desired needs. It is a decision-making process (often iterative), in which the basic sciences, mathematics, and the engineering sciences are applied to convert resources optimally to meet these stated needs (ABET, 2009).
1.4 THE SYSTEMS APPROACH
With the rapid technological advances made in every sphere of inquiry, engineers, planners, managers, decision makers, and even pure scientists realized that the complexity of real-world problems could not be handled by simply applying the traditional scientific method, which had its limitations. This is particularly true when dealing with social systems or engineering problems that have a social or human component. Indeed, if you look around for an engineering problem without the human factor, you would be hard pressed to find one. So then, where do we begin? Or better still, where should we begin? We will begin with a simple basic definition of the systems approach:
The systems approach represents a broad-based, systematic approach for problem solving and is particularly geared toward solving complex problems that involve systems. A system is a set of interrelated parts — components — that perform a number of functions to achieve common goals. Systems analysis is the application of the scientific method modified to capture the holistic nature of the real world in order to solve complex problems. In fact, the systems approach ought to be called the systemic approach; systemic in the sense that it offers systemic (holistic rather than piecemeal) as well as systematic (step-by-step rather than intuitive) guidelines for engineers to follow (Flood and Carson, 1993; Jackson, 2000).
Goals are desired end states, and operational statements of goals are called objectives that should be measurable (where possible) and attainable. Feedback and control are essential for the effective performance of a system. The development of objectives may in itself involve an iterative process. Objectives will generally suggest their own appropriate measures of effectiveness (MOEs). A MOE is a measurement of the degree to which each alternative action satisfies the objective. Measures of the benefits foregone or the opportunities lost for each of the alternatives are called measures of costs (MOC). MOCs are the consequences of decisions. A criterion relates the MOE to the MOC by stating a decision rule for selecting among several alternative actions whose costs and effectiveness have been determined. One particular type of criterion, a standard, is a fixed objective: the lowest (or highest) level of performance acceptable. In other words, a standard represents a cutoff point beyond which performance is rejected (Khisty and Lall, 2003). The following example will help you to understand the basic concepts.
1.5 STEPS IN SYSTEMS ANALYSIS
Some of the basic steps recommended for performing an analysis are:
Recognizing community problems and values
Establishing goals and defining the objectives
Establishing criteria
Designing alternative actions to achieve Step 2
Evaluating the alternative actions in terms of effectiveness and costs
Selecting an alternative action in keeping with the goals and objectives, criteria, standards, and value sets established, through iteration, until a satisfactory solution is reached
A simplified analysis process is illustrated in Figure 1.1, and the hierarchical interrelationships among values, goals, objectives, and criteria are shown in Figure 1.2.
1.6 CLASSIFICATION OF SYSTEMS
There is no standard classification scheme for systems. Boulding's (1956)hierarchy, ranked in an increasing order of complexity, was one of the first attempts at classification. Each level is said to include, in some way, the lower levels, but also to have its own emergent properties, although there is no scale of complexity attached to this hierarchy.
The most important distinction is between living and nonliving systems and the different types of relationship in the organizational connections involved within each hierarchy, including the lower hierarchies. Table 1.1 displays Boulding's hierarchy.
Boulding states, "One advantage of exhibiting a hierarchy of systems in this way is that it gives us some idea of the present gaps in both theoretical and empirical knowledge. Adequate theoretical models extend up to about the fourth level, and not much beyond." Since his remarks in 1956, significant progress has taken place in understanding systems, at nearly all levels, and new typologies have been published, based in part on Boulding's hierarchy.
1.7 SYSTEMS CHARACTERISTICS
During the past forty years, several authors have suggested basic considerations concerning systems thinking. Of all the proponents of systems,C. West Churchman (1968) has given us perhaps one of the simplest and yet the most understandable expositions of the subject. He outlines five basic considerations:
1. Objectives of the total system and, specifically, the measure of performance of the system itself
2. System environment
3. Resources of the system
4. Components of the system
5. Management of the system
These five considerations are not meant to be all-inclusive, but they capture most of the properties that engineers generally encounter in their practice. Let's discuss each of the points.
1. Objectives are those goals or ends that the system is working toward. Hence goal-seeking (or teleology) is a characteristic of systems. While the determination of objectives for mechanical systems is comparatively easy, those for human activity systems can be considerably more difficult. One must be cautious to distinguish between stated objectives and the real objectives of the system. For example, a student may say that her objective is to gain knowledge in order to master the subject being studied. Her real objective, in fact, simply may be to obtain good grades. In real life, objectives need to be operationalized, and to do this properly they need to be quantifiable, in some way or another, in order to measure the performance of the system. Quantification helps us to measure to what degree the system's objectives are being realized.
2. Environment constitutes all that is outside of the system. First, the environment includes all that lies outside of the system's control. Second, the environment also includes all that determines, in part at least, the manner of the system's performance.
3. Resources are all the means available to the system for the execution of the activities necessary for goal realization. Resources include all the things that the system can change and use to its own advantage. In human systems, one can also include the opportunities that are available to the system, besides human labor, money, and materials.
4. Components consist of the elements that accomplish the missions, jobs, or activities that the system has to perform to realize its objectives.
5. Management includes two basic functions. First, it is planning the system which involves all aspects of systems previously encountered, that is, its goals, environment, utilization of resources, and components and activities. Second, it is controlling the system which involves both the examination of the execution of plans as well as planning for change. Plans must be subject to periodic review and reevaluation because no plan can remain static throughout the life of a project. Associated with the planning and control function is the notion of information flow and feedback, often characteristic of cybernetic systems.
1.8 SYSTEMS ANALYSIS AND DECISION MAKING
We have described Churchman's systems approach for dealing with problems. You may notice that in contrast to the methods used in the pure sciences, the objectives of the systems approach is to recommend a course of action to the decision maker in addition to merely understanding the problem. Systems analysis is truly a continuous cycle of defining objectives, then designing alternative systems to achieve those objectives, evaluating the alternatives in terms of their effectiveness and cost, questioning the objectives and other assumptions underlying the analysis, seeking new alternatives and establishing new objectives, and the iteration goes on indefinitely. This cyclical process bears out the idea of the rational methodology to problems of choice.
(Continues…)
Excerpted from "Systems Engineering"
by .
Copyright © 2012 J. Ross Publishing.
Excerpted by permission of J. Ross Publishing, Inc..
All rights reserved. No part of this excerpt may be reproduced or reprinted without permission in writing from the publisher.
Excerpts are provided by Dial-A-Book Inc. solely for the personal use of visitors to this web site.
Table of Contents
CHAPTER 1 Mapping the Terrain of the Systems Approach,
CHAPTER 2 Problem Solving and Designing in Engineering and Planning,
CHAPTER 3 Basic Engineering Economics and Evaluation,
CHAPTER 4 Basic Microeconomics for Engineers and Planners,
CHAPTER 5 Principles of Probability: Part I — Review of Probability Theory,
CHAPTER 6 Principles of Probability: Part II — Random Variables and Probability Distributions,
CHAPTER 7 Principles of Probability: Part III — Joint Probability Functions and Correlated Variables,
CHAPTER 8 Principles Of Statistics: Part I — Estimation Of Statistical Parameters and Testing Validity of Distribution Functions,
CHAPTER 9 Principles of Statistics: Part II — Hypothesis Testing, Analysis of Variance, Regression, and Correlation Analysis,
CHAPTER 10 Basic Hard Systems Engineering: Part I,
CHAPTER 11 Basic Hard Systems Engineering: Part II,
CHAPTER 12 Systems Thinking,
CHAPTER 13 Systems Thinking: Case Studies,
CHAPTER 14 Sustainable Development, Sustainability, Engineering and Planning,
CHAPTER 15 Case Studies In Engineering and Planning for Sustainability,
Appendix,