Turfgrass Biology, Genetics, and Breeding / Edition 1 available in Hardcover
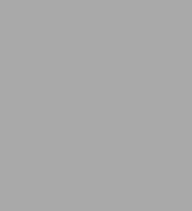
Turfgrass Biology, Genetics, and Breeding / Edition 1
- ISBN-10:
- 0471444103
- ISBN-13:
- 9780471444107
- Pub. Date:
- 01/30/2003
- Publisher:
- Wiley
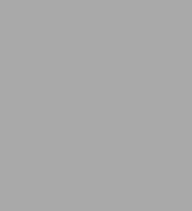
Turfgrass Biology, Genetics, and Breeding / Edition 1
Hardcover
Buy New
$151.95Overview
Product Details
ISBN-13: | 9780471444107 |
---|---|
Publisher: | Wiley |
Publication date: | 01/30/2003 |
Pages: | 384 |
Product dimensions: | 7.20(w) x 10.14(h) x 1.03(d) |
About the Author
RONNY R. DUNCAN, PhD, is a professor of Turfgrass Breeding and Stress Physiology in the Crop and Soil Sciences Department at the University of Georgia, Griffen. He's the author of Seashore Paspalum and Salt-Affected Turfgrass Sites, both published by Wiley.
Read an Excerpt
Turfgrass Biology, Genetics, and Breeding
John Wiley & Sons
ISBN: 0-471-44410-3Chapter One
Origins of the Turfgrasses
M.D. Casler and R.R. Duncan
Three types of selective forces act to modify populations of organisms (Darwin, 1875), including all turfgrass species. Natural selection is the process whereby those individuals best fitted to a particular environment, a range of fluctuating environments, or a human-imposed management system have the greatest survival rate or contribute the greatest numbers of viable progeny to succeeding generations. Unconscious selection is the process by which humans save the phenotypically most valuable or desirable individuals, or their seed, and destroy or ignore the less valuable or desirable individuals. This process allows humans to facilitate genetic changes insofar as genetic variation allows, without the need to define or predetermine selection criteria or potential correlated traits. Methodical selection comprises the forces that are applied by humans in their systematic attempts to create predetermined changes to populations. All three of these selective forces have acted and continue to act to create the worldwide pool of turfgrass germ plasm.
Turfgrasses are used in a range of highly artificial and contrived environments, many of which bear no similarity to natural environments in which turfgrasses evolved over many millennia. Turf environments are human-defined and are often highly stressful, typically combining a large number of biotic and abiotic stressfactors (Duncan and Carrow, 1999) that are seldom combined, or may be altogether absent, in natural habitats. Ironically, all turfgrasses arose from natural habitats, including mountain meadows, prairie and steppe grasslands, coastal plains, riparian and estuarine habitats, and many other habitats. Prior to human intervention and our discovery that perennial grasses can enhance our quality of life, these grasses served other purposes in the grand evolutionary scheme of Planet Earth.
NATURAL SELECTION
Perennial grasses coevolved with herbivores during the Miocene epoch. Fossil records suggest that evolution of the extensive North American grasslands was largely responsible for the great evolutionary advances in the horse, including increase in body size, strengthening of teeth, and loss of toes (Thomasson, 1979). Likewise, perennial grasses have evolved numerous defense mechanisms to survive and/or thrive under grazing pressure, including axillary meristems, rhizomes, stolons, trichomes, siliceous dentations, alkaloids, phenolic compounds, and associations with endophytic fungi (Casler et al., 1996). Many grasses have become dependent on herbivores-grazing pressure is often required for their survival (McNaughton, 1979). Perennial grasses respond to grazing by increasing their photosynthetic rate, leaf growth rate, and protein concentration, making them more desirable and nutritionally valuable to graziers (McNaughton et al., 1982).
The evolution of grasslands may have partially directed the evolution of humans, forcing the upright gait, the tool-using hand, and the heightened intellect required for survival in such a demanding habitat (Pohl, 1986). Whether grasses contributed to the prehistorical evolution of humans is highly debatable. However, it is scarcely debatable that perennial grasses are an integral component of the evolution of modern humans and our societies. Perennial grasses have evolved a large number of traits that collectively serve to make them useful for human enterprise and recreation. As human needs change, so too will turfgrasses, resulting in future coevolution with humans.
The evolution of perennial grasses generated tremendous inter- and intraspecific variability for adaptation. It requires little scientific expertise to observe and identify adaptive variation among some species, e.g., poor winter survival of many warm-season grasses in cold climates and poor summer survival of many cool-season grasses in warm climates. The tremendous differences among turfgrass species in adaptation to diverse climates and biotomes are illustrated throughout this text. What is less obvious to untrained observers is the tremendous variability that natural selection pressures have generated within species. Natural selection has resulted in ecotypic variability within all turfgrasses, providing the genetic foundation for all turfgrass cultivars that have been developed to date.
Most perennial turfgrass plants are highly heterozygous and originate from highly heterogeneous populations. These species are generally highly self-incompatible (with facultative apomicts as the obvious exceptions) and often exist as a polyploid series. Furthermore, many turfgrass species, as reviewed later in this text, freely exchange genes with their closest relatives due to similar genomic structures and frequent high levels of cross-compatibility. These characteristics, combined with the ever-present possibility of new mutations, act to create highly heterogeneous populations with huge numbers of potential genotypes and the possibility of creating new genotypes with each pollination event.
The natural environments in which these heterogeneous populations evolved are typically characterized by fluctuating environmental conditions, often including annual cycles of fluctuating temperature, humidity, precipitation, and sunlight. Furthermore, these geophysical and geoclimatic cycles are not constant, varying across decades and centuries (Ruzmaikin, 1999; Cai and Whetton, 2000; Cockell, 2000; Robertson et al., 2000). Climatic fluctuation creates natural selection pressures that vary in intensity and direction, resulting in populations with mixtures of interbreeding phenotypes favored by different environmental conditions. This process, a form of disruptive selection, maintains a large amount of genetic variation within populations.
Biotic Stresses
The high degree of uniformity, the presence of numerous predisposing abiotic stresses, the constant presence of litter and/or decomposing tissue, and the use of mechanical mowers in turfgrass environments often leads to disease epidemics and epiphytotics unlike those that occur in nature (Endo, 1972). Nevertheless, turfgrasses have coevolved with many disease-causing pathogens, resulting in alleles for resistance in many natural populations. Some turfgrasses, such as perennial ryegrass (Lolium perenne L.), have evolved loci that act in a gene-for-gene manner with highly virulent and variable pathogens, such as the rust fungi (Puccinia spp.) (Wilkins, 1978, 1991). In many other pathosystems, resistance is less obvious, often measured on a quantitative scale and likely controlled by loci with small individual effects (Casler and Pederson, 1996). Natural selection has also created morphological and chemical barriers that act as resistance mechanisms to various insects (Chamberlain and Evans, 1979; Lancashire et al., 1977).
Abiotic Stresses
The variability present within most perennial grasses has allowed them to colonize a large portion of the Earth's land mass. The Park Grass Experiments at Rothamstead, reviewed by Snaydon (1973, 1978), have shown that natural selection can create rapid genetic shifts in natural populations of perennial grasses. Natural populations of perennial grasses contain loci that confer resistance or tolerance to a wide array of abiotic stresses, often including opposite extremes of the same stress factor, such as soil pH or fertility. Less than 50 years were required to bring about dramatic changes in populations for limed vs. unlimed or high- vs. low-P soils. In some cases evolutionary changes to an environmental extreme resulted in a population unable to survive at the opposite environmental extreme. The infrequent cutting management that allowed surviving plants to undergo sexual reproduction, combined with human intervention to create a more-or-less constant environment, may have been partly responsible for these rapid changes.
Cold or freezing tolerance shows considerable variation among accessions of several turfgrass species. In perennial ryegrass, freezing tolerance of natural populations is closely associated with mean temperature of the coldest month at their site of origin (Humphreys and Eagles, 1988; Tcacenco et al., 1989). Crown height, as indicated by subcrown internode length, is inversely related to cold tolerance in perennial ryegrass (Wood and Cohen, 1984). In tall fescue (Festuca arundinacea Schreb.), genome size (amount of heterochromatin and frequency of repeated DNA sequences) was positively correlated with latitude of origin, suggesting that structural changes in DNA may play a role in environmental adaptation (Ceccarelli et al., 1992).
Natural variation exists for drought tolerance in species such as perennial ryegrass, which possess a wide geographic and climatic range. Accessions collected from consistently dry habitats have greater drought tolerance than commercial cultivars (Reed et al., 1987). Numerous drought-tolerance or drought-avoidance mechanisms have evolved in perennial ryegrass, including genetic variation for stomatal resistance (via differences in stomatal size and/or frequency), depth of epidermal ridging, leaf water conductance, and leaf osmotic potential (reviewed by Casler et al., 1996).
Tolerance to salinity has evolved naturally in numerous turfgrasses, including members of Agrostis, Festuca, Lolium, and Poa (Venables and Wilkins, 1978; Wu, 1981; Humphreys et al., 1986; Acharya et al., 1992). The evolution of salt tolerance in turfgrass has occurred in coastal marshes and rocky, alpine habitats. Natural selection pressure for salt tolerance drives selection coefficients, the rate at which alleles change in natural populations, and the eventual extent to which salt tolerance evolves (Ahmad et al., 1981; Wu, 1981). Natural populations can vary dramatically in salt tolerance across distances as little as 10 m. Salt tolerant genotypes of turfgrasses can evolve a form of salt dependence, as illustrated by greater increases in root and shoot growth under high- vs. low-saline growth conditions (Ashraf et al., 1986). A comprehensive listing of salinity tolerance levels across turf species is available (Carrow and Duncan, 1998). Seashore paspalum (Paspalum vaginatum Swatrz) has genetic variation for salinity tolerance varying from bermudagrass [Cynodon dactylon (L.) Pers.] tolerance levels to ocean-salt levels (Lee, 2000).
UNCONSCIOUS SELECTION
Domestication of Livestock and Grasses
Domestication is evolution under human influence (Harlan, 1975). In a strict sense, domesticated species should be phenotypically distinct from their wild forms, such that domesticated forms are clearly more useful to humans (Isaac, 1970). With one exception, perennial forage grasses are not domesticated; "wild" collections are generally phenotypically indistinct from cultivated forms. The single exception is Italian ryegrass (Lolium multiflorum Lam.), which was developed by unconscious selection prior to the twelfth century in the Lombardy and Piedmont plains of Italy (Beddows, 1953). Ryegrass (Lolium spp.) once existed as a "huge hybrid swarm" with perennial and Italian phenotypes representing opposite extremes of a continuum (Tyler et al., 1987). Hay harvesting, followed by reseeding with shattered seed, resulted in the tall, sparse-tillered, semiannual phenotype that has since been elevated to species status (Breese and Tyler, 1986).
The initial events in the domestication of turfgrasses likely were associated with the domestication of livestock for agriculture. Animals were herded, corralled, or tethered in tight groups, creating closely-grazed sods that may have enticed humans to create games of sport, skill, and strength (Roberts et al., 1992). Animals were kept close to homes to protect them from wild carnivores, resulting in grazed sods in close proximity to homesteads, perhaps leading to an evolution of human needs and desires for lawns and landscaping. Many graziers, even in the highly mechanized rural societies of contemporary industrialized countries, continue to use sheep and goats to maintain their home lawns.
The practice of herding animals, particularly if accompanied by a human desire to maintain a closely-grazed sod for long periods of time, set in motion selective forces unlike those that exist in nature. Human needs for uniformity and consistency of sward management put pressure on plants to survive under long periods of stress, pressured by stresses that may not have occurred in nature. Grazing pressure can create rapid shifts in the phenotype of grass populations, with phenotypic changes proportional to grazing intensity and duration (Brougham et al., 1960; Charles, 1964; Brougham and Harris, 1967). Indeed, perennial ryegrass appears specifically adapted to survive in association with grazers (Beddows, 1953; Breese, 1983) and is rarely found in natural habitats devoid of grazing pressure (Davies et al., 1973). Perennial ryegrass appears to have spread throughout the Mediterranean Basin in direct association with the development and spread of livestock agriculture via human migration (Balfourier et al., 2000).
Frequent defoliation of perennial grass populations results in genetic shifts toward shorter basal internodes, a more prostrate growth habit, higher tiller densities, later flowering dates, and greater longevity of individual plants (Casler et al., 1996). In some cases, long-term defoliation by either herbivores or mechanical means (constant and low mowing heights) can bring about such changes (Van Dijk, 1955). Frequent defoliation can lead to proliferation of genotypes that are extremely long-lived, with estimates of a single Holcus mollis L. clone covering a distance of 800 m (Harberd, 1967) and a single Festuca rubra L. clone over 1,000 years old (Harberd, 1961).
Human Ball Games and Grass
The genesis of human ball games was at least 5,000 years ago, dating to the Egyptian game of ninepins and the Greek form of hockey (Altham and Swanton, 1926). Soccer dates to China in the third century B.C. as a form of military training called 'Tsu Chu' (Green, 1953). According to Green, the expression Tsu Chu derives from Tsu, to kick the ball with feet, and Chu, a stuffed ball made of leather. Other forms of 'football' derive from the Romans about the same time (Marples, 1954). Early versions of football games were violent, undisciplined, and relatively rule-free, suggesting that grass playing fields were highly desirable for the participants' well-being. (Imagine playing modern rugby or American football on bare soil or gravel without modern protective equipment, medications, or antibiotics.)
Continues...
Excerpted from Turfgrass Biology, Genetics, and Breeding Excerpted by permission.
All rights reserved. No part of this excerpt may be reproduced or reprinted without permission in writing from the publisher.
Excerpts are provided by Dial-A-Book Inc. solely for the personal use of visitors to this web site.
Table of Contents
PART 1. INTRODUCTION.Origins of the Turfgrasses (M.D. Casler and R.R. Duncan).
PART 2. COOL-SEASON GRASSES.
Kentucky Bluegrass (D.R. Huff).
Annual Bluegrass (D.R. Huff).
Supina Bluegrass (S. Bughrara).
Texas Bluegrass (J.C. Read and S.J. Anderson).
Rough Bluegrass (R. Hurley).
Perennial Ryegrass (D. Thorogood).
Tall Fescue (W.A. Meyer and E. Watkins).
Fine-Leaved Festuca Species (B.A. Ruemmele, et al.).
Creeping Bentgrass (S.E. Warnke).
Colonial Bentgrass (B.A. Ruemmele).
Velvet Bentgrass (L. Brilman).
Three Minor Agrostis Species: Redtop, Highland Bentgrass, and Idaha Bentgrass (A.D. Brede and M.J. Sellman).
Hairgrasses (L. Brilman and E. Watkins).
PART 3. WARM-SEASON GRASSES.
Bermudagrass (C.M. Taliaferro).
Buffalograss (T.P. Riordan and S.J. Browning).
Zoysiagrasses (M.C. Engelke and S. Anderson).
Centipedegrass (W.W. Hanna and J. Liu).
Seashore Paspalum (R.R. Duncan).
St. Augustinegrass (P. Busey).
Bahiagrass (P. Busey).
Index.