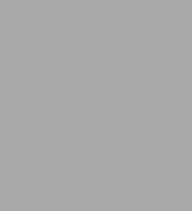
Conservation by Proxy: Indicator, Umbrella, Keystone, Flagship, and Other Surrogate Species
400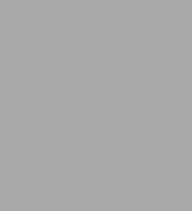
Conservation by Proxy: Indicator, Umbrella, Keystone, Flagship, and Other Surrogate Species
400eBook
Available on Compatible NOOK devices, the free NOOK App and in My Digital Library.
Related collections and offers
Overview
Synthesizing an immense body of literature, conservation biologist and field researcher Tim Caro offers systematic definitions of surrogate species concepts, explores biological theories that underlie them, considers how surrogate species are chosen, critically examines evidence for and against their utility, and makes recommendations for their continued use. The book
clarifies terminology and contrasts how different terms are used in the real world
considers the ecological, taxonomic, and political underpinnings of these shortcuts
identifies criteria that make for good surrogate species
outlines the circumstances where the application of the surrogate species concept shows promise
Conservation by Proxy is a benchmark reference that provides clear definitions and common understanding of the evidence and theory behind surrogate species. It is the first book to review and bring together literature on more than fifteen types of surrogate species, enabling us to assess their role in conservation and offering guidelines on how they can be used most effectively.
Product Details
ISBN-13: | 9781597269599 |
---|---|
Publisher: | Island Press |
Publication date: | 06/23/2010 |
Sold by: | Barnes & Noble |
Format: | eBook |
Pages: | 400 |
Sales rank: | 732,446 |
File size: | 6 MB |
About the Author
Read an Excerpt
Conservation by Proxy
Indicator, Umbrella, Keystone, Flagship, and Other Surrogate Species
By Tim Caro, Sheila Girling
ISLAND PRESS
Copyright © 2010 Island PressAll rights reserved.
ISBN: 978-1-59726-959-9
CHAPTER 1
Buzzwords in Conservation Biology
Shortcuts
The goal of conservation biology is to stop or delay the extinction of plant and animal populations and to prevent or slow habitat destruction. As populations, species, and habitats are under threat in so many places, we are forced to make difficult decisions about where to focus conservation attention. Ideally, detailed study should precede important decisions, especially those that have long-term ramifications for conservation, but four factors prohibit this: the complexity of nature prevents accurate appraisal of all its aspects, the scale of the biodiversity crisis is vast, political decisions must be made rapidly, and there is a severe shortage of funds. Consequently, conservation scientists are compelled to take shortcuts to identify and solve problems. These involve using satellite imagery to monitor environmental change, modeling population responses to anthropogenic pressure, interviewing people about their activities, garnering expert opinion about species' distributions and the threats they face, and monitoring subsets of species. Subsets may act as proxies for the presence of others, and may help us in deciding where to set up protected areas, in measuring plant and animal community responses to anthropogenic change, and in raising conservation awareness. They are called surrogate species or surrogate taxa, which I define as "species that are used to represent other species or aspects of the environment to attain a conservation objective" (see also Wiens et al. 2008).
Surrogates may be species that represent the whole pool of species, or those that represent subsets of the species pool, or they may be species-groups that represent the species pool (see Fig. 1-1). All of these fall under the rubric of surrogate species in this book. Broadly, surrogates are likely to be most useful when the number of species being protected or monitored is uncertain, or the spatial extent of the task is intermediate in size (Wiens et al. 2008). When the area or number of species is very small, individual species can sometimes be considered one by one, and when the area or number of species is very large, surrogate species may be unable to represent the variety of taxa or habitats present and so may not be helpful. Spatial scale is thus very important in the science of surrogate species.
At its heart, the surrogate species concept relies on extrapolation, from group A to group B, from area A to area B, from subgroup to group, from smaller to larger scale, sample to inventory, habitat to inventory, and so on (Hammond 1995). The key issue is whether such extrapolations are valid. Until we know this, the surrogate species concept is a risky gambit because we may be making unreliable approximations of the larger picture. Nevertheless, perhaps because surrogate terms are catchy shorthand expressions and have now become conservation buzzwords, unreliable and ill-conceived surrogate species continue to be proposed in conservation workshops, in meetings, and in the literature, potentially affecting important management decisions without careful thought as to what such terms really signify in nature and without considering the burden that they carry for conserving species and habitats. Moreover, surrogate concepts are often interchanged, elided, or just used incorrectly, generating a loose terminology that is confusing to laypersons, wildlife managers, and conservation scientists alike. In short, buzzwords in conservation are useful only if they are clearly defined, address clear objectives, and prove themselves to be effective.
I start this chapter by introducing issues of biodiversity and scale, then outline the variety of ways surrogate species are used in conservation, and present five different problems in their application. This chapter provides a sketch of how surrogate concepts are brought into play and misused in conservation science.
Biodiversity
Usage
Biological diversity or biodiversity (Wilson 1992; Harper & Hawksworth 1995) "means the variability among living organisms from all sources including, inter alia, terrestrial, marine, and other aquatic systems, and the ecological complexes of which they are part; this includes diversity within species, between species, and of ecosystems" (Heywood 1995, 8; see also OTA 1987; Hunter 1996; Hubbell 2001; Groves et al. 2002; and Magurran 2004). The concept captures variability in biological systems from genes to communities but in practice centers on species richness (i.e., the number of species present) in an area. Species richness is reasonably precise (at least for animals), relatively easy to measure, and is generally assumed to play a positive role in ecosystem dynamics. Some people erroneously equate species richness with a diversity (i.e., the number of species in a homogeneous habitat), but it can also refer to ß diversity, the difference in composition of species between habitats located in close proximity in the same landscape (also referred to as species turnover or community dissimilarity, Whittaker 1960; Su et al. 2004). Measures of ß diversity can be quantified in several ways, using Jaccard's coefficient or percentage similarity, for example. Alpha diversity increases with the size of the area sampled, whereas ß diversity declines because fewer new species are encountered; γ diversity refers to the total number of species in a given region.
Biodiversity is also measured using combinatorial measures of richness and abundance (Maclaurin & Sterelny 2008), allowing species community structure and composition to be assessed using measures such as the Q statistic, Simpson's index, and Generalized Dissimilarity Modeling (Ferrier et al. 2007).
Biodiversity is used in other senses as well. Endemic species have relatively small geographic ranges (e.g., an average of 64,561 km2 for 147 endemic Mexican mammals versus 427,183 km2 for 314 nonendemics, Ceballos et al. 1998). They may be restricted to an ecoregion, such as mangrove forests, or to a country—bonobos are endemic to the Democratic Republic of Congo, for instance. Species with narrow geographic ranges are especially susceptible to extinction from disease, invasive species, sustained habitat degradation, climate change, or political instability (Usher 1986; Pimmet al. 1995).
Rarity is a component of biodiversity, too. It is important in conservation because rare species are prone to extinction. Rarity can be measured in three orthogonal ways—a wide or narrow geographic distribution, a broad or restricted habitat specificity, or a local population size that is somewhere large or everywhere small (Rabinowitz et al. 1986). These can be combined together to produce seven different forms of rarity and one form of commonness, although in practice rare species are frequently defined by their limited geographic distribution—often as the lowest quartile of species based on their representation on a geographic grid (Gaston 1994; Flather & Sieg 2007)—so in this sense they are similar to narrow endemics. They are also defined as species with absolutely or relatively small population sizes (Andelman & Fagan 2000). Other definitions include habitat specialists and, confusingly, threatened species, although these need not be synonymous (Freitag & van Jaarsveld 1997), as well as combinations of measures (Cofre & Marquet 1999; Marcot & Flather 2007). In ecological surveys, rare species can denote species of which only a single individual is recorded (Novotny & Basset 2000), or those found at only one site (Longino et al. 2002)—such rarity can be more apparent than real because it is affected by sampling effort.
Threatened species are yet another aspect of biodiversity. Threat refers to processes that drive loss of biodiversity, such as logging and mining. It can be considered in terms of exposure, or probability of a threatening process affecting an area, or intensity or magnitude, or the impact or outcome of the threat. Vulnerability is a closely related term that often refers to species and populations but also relates to the area of occupancy (Wilson et al. 2005). Conventionally, the extent to which a species is vulnerable is measured using the International Union of Nature and Natural Resources (IUCN) categorization of threatened and endangered species (see Table 1-1a) in which species are ascribed categories using several criteria (see Table 1-1b); sometimes these categories are assigned ordinal measures (e.g., Rey Benayas & de la Montana 2003). The method is transparent and can be updated with new information (IUCN 2008), and changes in species categorization over time enable trends in extinction risk to be tracked (e.g., Butchart et al. 2005). Threatened species can also be defined in terms of combinatorial measures of population size and speed of decline, independent measures including the Food and Agriculture Organization's data on annual percentage loss of forests over set time intervals, human population density and rates of increase, whether or not species are featured on various other endangered lists, or even the absence of protected areas within a country (Millsap et al. 1990; Master 1991; Sisk et al. 1994; Foose & van Strien 1997).
Documentation
The way in which species numbers are tallied affects the total number of endemic, rare, or threatened species that are recorded. When species in an area are counted using geographic range maps, the sum is higher than that based on surveys because species are not found everywhere throughout their geographic range (Rondinini et al. 2005). North American birds are detected on average at only 40.5 percent of sites within their mapped ranges during a single year survey, for instance. Species with low range occupancy are those with low average densities and narrow habitat requirements—species occupying high elevations, for example (Hurlbert & White 2005). Combining survey and range-map distributions conflates measures of differing resolution.
Additionally, estimates of species numbers depend on scientific expertise. Well-known groups such as birds and vascular plants can be identified by nonspecialists, but others such as arthropods may be identified only to morphospecies and thus be subject to biases caused by sexual dimorphism and phenotypic developmental changes that will boost species totals, or by cryptic species (different species that look morphologically similar) that will diminish species totals.
Remarkable Species
Simple species richness at a site misses the important concept of taxonomic distinctiveness in which distantly related species are thought to be worthy of more conservation effort than closely related species. (Figure 1-2 shows how taxonomic distinctiveness can be calculated.) In triage operations where only a limited number of species can be "saved," heuristic models have been put forward that maximize the number of speciation events (Vane-Wright et al. 1991) or branch lengths (Faith 1992) within a clade, as opposed to number of species. These demand rather detailed cladograms and knowledge of character state changes that are unavailable for most taxa and so have yet to be formally used in prioritizing sites of high biodiversity (Mace et al. 2003). Where proposals to conserve species richness or taxonomic distinctiveness have been pitted against each other (e.g., Virolainen et al. 2001), outcomes can differ enormously because high species totals often arise from recent adaptive radiations in which species are closely related rather than taxonomically distinct. This discrepancy means the criterion on which reserves are chosen has ramifications for species representation (Forest et al. 2007).
Scale
Scale is an important issue in ecology because associations between species richness and altitude, latitude, productivity, and other variables depend on the scale at which species richness is measured (Levin 1992; Schneider 1994; Poiani et al. 2000; Rahbek 2005). For instance, the extent to which hummingbird species richness in South America is predicted by latitude and topography varies with size of grid cell (see Fig. 1-3). In conservation, scale is vital for at least three reasons. First, identifying patterns of biodiversity at a global or continental scale is important for determining which biogeographic units need conservation attention, but practical decisions about land use occur at smaller national, regional, or local levels. In the words of Vane-Wright and colleagues as far back as 1991:
[ILLUSTRATION OMITTED]
Identification of whole countries, geographic regions, islands or large grid squares as critical faunas or floras is a basic step, but such units rarely represent practically conservable areas in terms of current economic resources. Furthermore, within critical areas, all species of interest rarely coexist at single localities. It is therefore necessary to undertake further rounds of analysis, within each priority region, to develop effective action plans for conservation. (249)
The second reason for the importance of scale is empirical: some studies indicate that biodiversity is associated closely across taxa at one scale of measurement but not at another, and further studies show that congruency between different biodiversity metrics can be differentially affected by scale. The general consensus is that patterns of biodiversity correspond less at finer scales of resolution (Reid 1998; Garson et al. 2002; Wolters et al. 2006). For example, species richness (Rahbek & Graves 2001) and forest destruction, a proxy for threatened species (Gaston & Blackburn 1996), both increase toward the tropics so these variables are likely to be associated at a global but not at a national scale. Effects of scale vary according to the measure of biodiversity used. In sub-Saharan Africa, grid cell sizes of sizes 1 × 1° up through 8 × 8° show high levels of coincidence in specifying the five (or ten) top-priority grid cells when measures of richness of rare mammal species, mammal species richness, and richness of endangered mammals are considered. There is little concordance between grid squares, however, if complementary species richness is at issue (Larsen & Rahbek 2003). Complementarity refers here to choosing grid squares on the basis of their holding the rarest species of mammal, then adding on squares holding the next rarest species of mammal, and so on—the difficulty being that species that are rare based on small grid cells may not be considered rare when based on large grid squares (Erasmus et al. 1999).
More generally, scale is comprised of two components—grain, the size of the observational unit; and extent, the size of the entire study area. Examining these independently, Hess and colleagues (2006) collated data from the mid-Atlantic and Pacific Northwest regions of the United States on amphibian, bird, butterfly, freshwater fish, freshwater mussel, mammal, and reptile species richness at four levels: hexagons (~649 km2), subecoregions (3,800–34,000 km2), ecoregions (8,300–79,000 km2), and geographic regions (315,000–426,000 km2). They examined correlations with varying extent of analysis (grain held constant at the hexagon) and varying grain (extent held constant at the region). The degree to which one taxon correlated with the remaining six taxa combined varied enormously by all three factors—grain, extent, and region of the United States from–0.93 (considering the smallest subecoregion value for the hexagon grain in the mid-Atlantic for mammals) to +0.96 (largest subecoregion value for the hexagon grain in the Pacific Northwest for mammals; see Fig. 1-4). Scale dependency therefore forces us to decide whether we want to maximize species richness at a small scale in our backyards or the county, or in the state, or at a large scale in a country, or even the entire globe (Gardenfors 2001), and scale-dependent differences in congruency dictate that the surrogate taxa by which we might measure this biodiversity will differ, too (Bohring-Gaese 1997).
The third reason for considering scale is that the effects of disturbance are scale-dependent. This is because α and ß diversity increase with spatial scale at a faster rate in undisturbed habitat than in disturbed habitat because of reduced habitat heterogeneity in the former and abridged spatial autocorrelation of diversity following disturbance (Dumbrell et al. 2008). This results in complex scale-dependent and taxonomic effects. For example, birds show greater species diversity in disturbed than undisturbed forests at a large scale but lower species diversity in disturbed as opposed to undisturbed forest at a small scale. In contrast, butterflies show lower species diversity in disturbed forests at a large scale but higher diversity there at a small scale (Hamer & Hill 2000; Hill & Hamer 2004). Consequently, effects of habitat disturbance should not be evaluated at a single spatial scale.
(Continues...)
Excerpted from Conservation by Proxy by Tim Caro, Sheila Girling. Copyright © 2010 Island Press. Excerpted by permission of ISLAND PRESS.
All rights reserved. No part of this excerpt may be reproduced or reprinted without permission in writing from the publisher.
Excerpts are provided by Dial-A-Book Inc. solely for the personal use of visitors to this web site.
Table of Contents
PrefacePART I. Introduction
Chapter 1. Buzzwords in Conservation Biology
- Shortcuts
- Biodiversity
- Scale
- Surrogate species in systematic conservation
- Difficulties in surrogate typology
- Summary
PART II. Distribution of Biodiversity
Chapter 2. Species Indicators of Biodiversity at a Large Scale
- A big picture
- Congruency of species richness
- Congruency of endemism
- Congruency of rarity
- Congruency of threatened species
- Complementarity and congruency
- Concordance between measures of biodiversity
- Biodiversity distribution and protected areas
- Practical application
- Summary
PART III. Reserve Site Selection
Chapter 3. Species Indicators of Biodiversity in Reserve Selection
- A smaller scale
- Cross-taxon congruence of species richness
- With-taxon congruence of species richness
- Congruency of endemism, congruency of rarity, and congruency of threatened species
- Concordance between measures of biodiversity
- Congruency of complementarity
- Protected area coverage
- Marine reserve prioritization
- Environmental surrogates
- Practical issues
- Summary
PART IV. Reserve Design and management
Chapter 4. Umbrella Species and Landscape Species
- Three conservation goals
- Lambeck's insight
- Umbrella species by taxon
- Choosing an appropriate umbrella species
- Problems with umbrella species
- Management implications
- Landscape species
- Summary
Chapter 5. Keystone, Engineering and Foundation Species
- The keystone species concept
- Ecosystem engineers
- Foundation species
- Management issues
- Summary
PART V. Species Indicators of Anthropegenic Change
Chapter 6. Environmental Indicator Species
- Ecosystem health and biological integrity
- Environmental indicators
- Examples of the uses of environmental indicator species
- Proliferation and obfuscation of terms
- Summary
Chapter 7. Ecological Disturbance Indicator Species
- Effects of disturbance
- Proposed criteria for indicator species
- Single species and species-groups as indicators of disturbance
- Examples of the use of species-groups in documenting effects of land-use change
- Changes in populations over time
- Determining the number of species-groups
- Management pointers
- Summary
Chapter 8. Cross-taxon Response Indicator Species
- Habitat alteration
- Fora for cross-taxon-response indicator species
- Intraguild-response indicator species
- Population changes
- Management indicator species
- Early warnings
- Substitute species
- Problems with cross-taxon-response indicator species
- Summary
PART VI. Promoting Conservation
Chapter 9. Flagship Species
- Characteristics of flagship species
- Multiple objectives
- Are flagship species successful?
- Qualities of flagship species
- Iconic species
- What's next?
- Summary
PART VII. Summary of Concepts and Cost-Effectiveness
Chapter 10. Surrogate Species in the Real World
- Surrogate categories
- Synopsis
- Distribution of biodiversity
- Reserve site selection
- Reserve design and management
- Species indicators of anthropogenic change
- Promoting conservation
- Wrap-up
- Summary
References
Scientific Names of Species Mentioned in the Text
Subject
Index