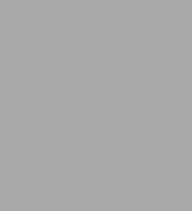
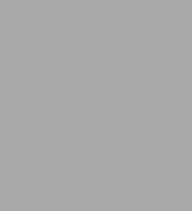
eBook
Available on Compatible NOOK devices, the free NOOK App and in My Digital Library.
Related collections and offers
Overview
The diesel locomotive sent shock waves through rigid corporate cultures and staid government regulators. For some, the new technology promised to be a source of enormous profits; for others, the railroad industry seemed a threat to their very livelihoods. Evolution of the American Diesel Locomotive introduces the reader to the important technological advances that gave rise to diesel engines, examining not only their impact on locomotive design, but also their impact on the economic and social landscapes. J. Parker Lamb describes the development of these technologies, allowing the reader to fully understand how they were integrated and formed a commercially successful locomotive. Like its companion volume, Perfecting the American Steam Locomotive (IUP, 2003), this book emphasizes the role of the leading engineers whose innovations paved the way for critical breakthroughs. Rail fans will appreciate this authoritative work.
“A host of books and articles have touched on various aspects of this ongoing story over the years, but none tell the story with the completeness and superb clarity found here.” —Michigan Railfan
“Lamb provides the reader with detailed descriptions of every generation of diesel locomotive along with a generous supply of excellent photographs.” —Technology and Culture
Product Details
ISBN-13: | 9780253027986 |
---|---|
Publisher: | Indiana University Press |
Publication date: | 12/22/2021 |
Series: | Railroads Past and Present |
Sold by: | Barnes & Noble |
Format: | eBook |
Pages: | 184 |
Sales rank: | 951,520 |
File size: | 22 MB |
Note: | This product may take a few minutes to download. |
Age Range: | 18 Years |
About the Author
J. Parker Lamb retired in 2001, after a 42-year career as a practicing engineer and university instructor, to pursue his life-long interest in the history of American technology with an emphasis on railroad developments. He has authored four books, including Perfecting the American Steam Locomotive (IUP, 2003). He lives in Austin, Texas.
Read an Excerpt
Evolution of the American Diesel Locomotive
By J. Parker Lamb
Indiana University Press
Copyright © 2007 J. Parker LambAll rights reserved.
ISBN: 978-0-253-02798-6
CHAPTER 1
PRECURSOR TECHNOLOGIES
Internal Combustion Engines
The transformation of thermal energy into mechanical power using a piston-cylinder configuration was one of the fundamental concepts brought to practicality during the Industrial Revolution of the nineteenth century. In these machines, piston movement is produced by a working fluid that becomes highly energetic through a combustion process. In the intervening centuries, two major types of reciprocating heat power machines have seen widespread use. The earliest type was the steam engine in which the working fluid (water) receives its thermal energy outside the power cylinder (in a boiler). A half-century later, the level of technological development had progressed to the point where a second type of reciprocating engine was possible. In this design, the working fluid (air) undergoes an explosive combustion process inside the power cylinder. Hence the common names for these two designs are based on whether the combustion occurs inside or outside the cylinder.
The first internal combustion engine concept was proposed in 1862 by Alphonse-Eugène Beau de Rochas, who was later issued a French patent for a generic internal ignition engine, although no actual machine was ever built. Even at this early stage, theoretical calculations showed that such an engine would have a much higher thermal efficiency than a conventional steam engine. This efficiency represents the proportion of thermal energy in the working fluid that is converted to mechanical work.
Credit for building the first working machine belongs to Nikolaus August Otto and his partner, Eugen Langen of Cologne, Germany. Unaware of de Rochas's patent, they developed and demonstrated a single-cylinder spark-ignition engine at the Paris Exhibition in 1867. Otto and Langen then formed a company to produce these engines, and by 1877 they had received an American patent and begun marketing them in the United States. The scientific world has given Otto's name to the subsequent design of all spark-ignition machines (i.e., Otto cycle engines).
Another pioneer in improving the spark engine was Gottlieb Daimler, who was superintendent of Otto's engine works in Germany. In 1879 he patented a multi-cylinder machine with a crankshaft, and he soon teamed up with Wilhelm Maybach to produce the first commercially successful automotive engine. Another German inventor, Carl Benz, perfected a system for precise timing of ignition arcs from spark plugs. Of course, Daimler and Benz would later form a German automotive company whose successors are still in existence. Some estimate of the gestation period of engine technology can be made by observing that, at the beginning of the twentieth century, after over 52,000 steam locomotives had been produced in the United States, there were only 936 American automobiles using spark-ignition engines.
A second major type of IC engine was originated in the 1890s by French-born Rudolf Diesel, who had received a doctoral degree from the engineering school in Munich in 1880. After some years of working on ice-making machines, his attention turned to the elimination of electrically powered spark plugs in the Otto engine. Diesel's concept involved compressing the intake air to such a high pressure and temperature that, when droplets of fuel were sprayed into the cylinder, a spontaneous combustion would occur. His theoretical calculations demonstrated that the higher temperatures and pressures inside the cylinder would produce a thermal efficiency even larger than that of the Otto engine.
Early successes of the compression engine led to great wealth and acclaim for Diesel. For example, in 1897 St. Louis brewer Adolphus Busch traveled to Germany and negotiated the sale of American rights for the manufacture of his engine. The Busch-Sulzer Brothers Diesel Engine Company began production in 1910. Dr. Diesel made two trips to America in 1904 to visit the St. Louis Exposition, where he met with Busch and Edward H. Harriman of the Union Pacific. Some reports suggest that Diesel convinced Harriman to construct an experimental diesel locomotive. Although the trade press announced the testing, no further information was ever published. On his 1912 trip, Diesel met with Thomas A. Edison and other luminaries and gave an address to the American Society of Mechanical Engineers in which he chided Americans for being too timid in exploiting the advantages of his new engine concept. Had he lived a normal life span, he would have been more than vindicated, but the work of this eccentric genius was cut short in 1913 when, in poor health and despondent over recent business failures, he disappeared during a nighttime ferry crossing of the English Channel (a probable suicide). Subsequent development of the compression-ignition engine lagged far behind the Otto design because the former required a level of materials development and manufacturing precision that was still two decades in the future.
Because these two IC engine designs lie at the heart of diesel locomotive evolution, it is desirable to describe some thermodynamic features of each, using a pressure-volume diagram (P-V) to depict schematically the physical processes occurring inside the cylinder. In chart 1.1, which illustrates the operation of a spark-ignition engine, the top-most position of the piston is known as top dead center (TDC), and the bottom limit is the bottom dead center (BDC). The volume being measured on the horizontal scale is the space above the top of the piston at each instant. Each passage of the piston between top and bottom is known as a stroke. The directional arrows indicate two strokes in each direction. Thus this sequence of operations is known as a four-stroke cycle Otto engine and more commonly as a four-cycle Otto engine.
The diagram identifies six events:
1 to A Intake stroke to pull air into the cylinder (intake valves open).
A to B Compression stroke to raise pressure and temperature of fuel-laden air.
B to C Spark ignition to further energize the fuel-air mixture.
C to D Power stroke to rotate crankshaft.
D to A Pressure decreases as exhaust valves open.
A to 1 Scavenge stroke to remove combustion gas from cylinder.
The four-stroke cycle requires two revolutions of the crankshaft for each power stroke. However, many engine designs are able to eliminate the first and last processes (represented by the dashed line in chart 1.1) and thus produce a two-stroke cycle engine. The applications of both designs to locomotive engines will be discussed later.
A similar P-V diagram of Diesel's original cycle is presented in chart 1.2a. The general shapes of the two diagrams differ only between B-C, which depicts a constant pressure ignition. Also, it must be remembered that the maximum pressure in a diesel cycle can be at least two times higher than that in an Otto cycle. Moreover, the compression ratio (volume at BDC divided by that at TDC) is also much larger for the diesel cycle. While this diagram displays the operation of an early low-rpm diesel, one must represent a contemporary high-speed engine using some aspects of the Otto cycle, as shown in chart 1.2b, where it is seen that the ignition begins during a short period of constant volume near TDC. This is often denoted as a mixed cycle or mixed diesel cycle.
A major caveat in these P-V diagrams is that they represent idealized conditions. In particular, the sharp corners at the intersection of two processes would certainly not be realistic. Thus a measured pressure variation inside the cylinder would display rounded corners at these intersection points. However, the simple theoretical depictions are effective as indicators of engine characteristics.
Electrical Machinery
The basic principle by which an electric motor operates is magnetism, first discovered by the English physicist Michael Faraday in the 1820s. Although some materials are naturally and permanently magnetic, he showed that an electric current passing through a conducting material would also produce a surrounding electromagnetic field. An important property of magnets is their polarity (normally called north and south poles and noted as + or -). Magnets having the same polarity will repel one another whereas opposing polarities will attract. Soon after Faraday's discoveries, Joseph Henry, a college instructor in Albany, New York, developed a practical electromagnet and used it to power an experimental motor that employed attraction and repulsion to produce a reciprocating motion that was then transformed into rotating motion (just as in an early steam engine).
The first electric motors of significance were based on the current produced by a metal-acid battery, the first electrical storage device. Batteries operate through an electrochemical action that occurs when metal electrodes react with the surrounding acid. This results in a steady migration of negatively charged subatomic particles (electrons) from one side of the battery to the other, thus creating a voltage difference and consequent current flow between anode and cathode (+ and - electrodes). Since battery current is steady and unidirectional, it was designated as direct current (DC). Around 1840 Robert Davidson, an electrical inventor in Aberdeen, Scotland, completed a 5-ton locomotive powered by 40 zinc-iron sulfuric acid batteries, while the pinnacle of battery-powered trains came in 1851 when Dr. Charles G. Page constructed a 16-hp locomotive powered by 100 battery cells. The reciprocating action of the magnets drove a flywheel that allowed the train to reach 19 mph on a 5-mile line between Washington, D.C., and Bladensburg, Maryland.
The first practical DC motor was built in 1860 by Italian engineer Antonio Pacinotti utilizing an unusual ring armature. An even more important discovery was made in 1873 when Zénobe Théophile Gramme of Belgium demonstrated that the components of a DC motor and a DC generator were identical. In one case the machine received mechanical power (shaft) and produced a DC output whereas, if it received DC input, it produced mechanical power via a rotating shaft.
The sketch in chart 1.3 illustrates the rudimentary elements of a DC generator. Between the two magnetic poles (N and S) there exists an invisible electromagnetic force field. When a conducting wire passes through this field at a certain orientation, it is said to be cutting lines of magnetic flux (force). The result is an electric current in the conductor wire. By mounting the conducting loop on a rotating shaft (armature), one can transmit the generated current away from the machine using a commutator, illustrated by the split ring and the two electric brushes (rubbing contacts). Note that this is a simple two-pole machine with two segments in the commutator ring. Most traction motors have four poles, but other applications may have more. In addition, industrial-sized electromagnets generally consist of metal slabs wrapped by coils of wire known as field coils or field windings.
With the development of reliable DC motors, the era of electric locomotives began in 1879 when Dr. Ernst Werner von Siemens built a demonstration locomotive for the Berlin Exhibition. Running on a half-meter gauge track, the tiny train hauled 100,000 people during the exhibition. This demonstration mirrored a similar event almost 50 years earlier (1831) when Matthias Baldwin demonstrated his first steam locomotive with a miniature train operating inside a large museum building in Philadelphia. Three years after Siemens's demonstration in Berlin, Thomas Edison laid 1,400 feet of track at his Menlo Park, New Jersey, laboratories and developed a small DC locomotive that pulled two cars at 40 mph.
Within a few decades it became obvious that steam-powered commuter and elevated lines were producing widespread urban pollution, and there began a national effort to utilize these newly developed electric trains, which were both cleaner and quieter than smoke-belching steamers (even if they were tiny 0-4-2 Forney tank engines). Frank J. Sprague was one of the early engineering leaders of electric-powered railway equipment. In 1885 he developed a prototype for an electric-powered train using elevated trackage of the Manhattan Railway. Three years later, he constructed the Richmond Union Passenger Railway, the nation's first all-electric street rail system. He also developed the first front-hung traction motors mounted on a pivoted truck and geared to driving axle, and he later invented a method of coupling two electrically driven cars under one controller (the multiple unit connection).
Finally in 1894 General Electric's Lynn, Massachusetts, plant constructed the first power unit designed for regular railroad service, an experimental single-truck 30-ton model with traction motors on each axle. It was exhibited at the World's Colombian Exhibition in Chicago in 1896 and then put to work for an industrial railroad in New Haven, Connecticut. The next GE model was the nation's first steeple cab design, a 35-ton B-B unit that used four 500-volt DC traction motors to produce a drawbar pull of 14,000 pounds. It was eventually sold to an industrial mill and used until 1964!
As DC propulsion advanced, the proper method of controlling traction motors for varying speed and varying loads became important. The physical basis for motor control begins with the relationship between voltage, current, and resistance. This can be written as
Voltage = Current × Resistance
where current is measured in amperes and resistance in ohms. This is known as Ohm's Law for circuits and can be applied to an entire circuit or to any element within the circuit. Symbolically this can be written as
E = I R
Moreover, electric power can be expressed as
Power = Voltage × Current
or
P = E I = I2 R
Power is measured in volt-amperes, usually kilovolt-amperes (kVa). Power is also much more dependent on current levels than voltage.
The DC motor's two components, armature and field coil, have different electrical resistances. Moreover, there are two choices for connecting these components, as shown in chart 1.4. In a DC-series motor they are connected end to end, while in a DC-shunt motor they are connected in parallel. For a series motor, the total resistance of the two elements is merely the sum of the component resistances, and therefore, according to Ohm's Law, the voltage across each element will be different, although electrical continuity requires that the current through each element be the same. In contrast, with a parallel circuit, the full voltage acts across each component, thus producing different currents through each part, depending on its resistance.
Early experimentation showed that the DC-series arrangement was the most desirable for traction motor applications. As the load (current) on the motor increases, the magnetic field strength automatically increases, and the result is an increase in torque with a decrease in speed. Consequently, the series motor tends to adjust itself readily to the varying tractive effort required to accelerate and decelerate a train. In addition, further control can be obtained by grouping DC -series motors themselves in either series or parallel circuits or even in a hybrid series-parallel arrangement. These three circuits are depicted in chart 1.5, where four traction motors receive power from a generator. In one circuit, all motors are in series and therefore each operates on one-fourth of the total voltage of the generator. In the full parallel circuit, the total voltage of the generator is imposed on each motor, while the hybrid circuit allows each pair of motors to operate on half of the total voltage. For early locomotives, the series circuit was used for starting, with transition to the series-parallel at intermediate speeds and to the full parallel at high speeds. Moreover, the transition was a manual two-handed procedure. Later machines included a fully automatic transition control, while most modern DC machines use only two motor circuits, parallel-shunt and full parallel, where the former is a modification of the series-parallel connection in which part of the current is shunted from the field to the armature to increase power.
While General Electric surged ahead of everyone else in developing DC machinery after the 1880s, its major rival became engaged in a crusade led by its determined owner, George Westinghouse, who strongly favored the newer form of electricity, alternating current (AC). As its name implies, the current and voltage in an AC system change direction many times each second, and thus AC is characterized by an oscillation frequency measured in cycles per second (cps). One cps is denoted as one Hertz in contemporary literature, while one Megahertz equals one million cycles per second.
(Continues...)
Excerpted from Evolution of the American Diesel Locomotive by J. Parker Lamb. Copyright © 2007 J. Parker Lamb. Excerpted by permission of Indiana University Press.
All rights reserved. No part of this excerpt may be reproduced or reprinted without permission in writing from the publisher.
Excerpts are provided by Dial-A-Book Inc. solely for the personal use of visitors to this web site.
Table of Contents
Preface
Chapter 1 Precursor Technologies
Chapter 2 Self-propelled Coaches
Chapter 3 The Diesel Climbs Aboard
Chapter 4 Streamlined Trains
Chapter 5 Developments Beyond La Grange
Chapter 6 Alco Rebounds
Chapter 7 Postwar Shakeout
Chapter 8 Road Switchers Take Over
Chapter 9 A Monopolized Market
Chapter 10 Special Purpose Designs
Chapter 10 New Heights for Diesel Power
Chapter 12 Recent Developments
Chapter 13 The Diesel Century in Perspective
References
Index
What People are Saying About This
"Lamb's book is . . . an important contribution to railroad technological history. The book's strength is the author's mastery of the mechanical details, which he presents in a straightforward style."--(Mark Reutter, editor of Railroad History)