Phosphorus-containing (co)polymers are gaining wide appeal for many uses, from healthcare and medicine to energy and environmental applications. Phosphorus-Based Polymers is the first book dedicated to this topic and provides a comprehensive overview of the different polymers and their uses.
The first part of the book covers the synthesis and polymerisation of different phosphorus containing systems including phosphorus containing (meth)acrylate, (meth)acrylamide and viny or allyl monomers, as well as vinyl phosphonic acid, 2-methacryloyloxyethyl phosphorylcholine, poly(phosphoesters)and polyphosphazenes. The second part of the book contains specific chapters detailing different applications such as biomedical applications in dental materials, tissue engineering and drug delivery, metal complexation for anti-corrosion and wastewater purification materials, fire retardant additives and fuel cell membranes.
Written by expert researchers in the chemistry of phosphorus-containing polymers, this book is suitable for academic and industrial researchers interested in polymer and materials synthesis as well as their applications.
Phosphorus-containing (co)polymers are gaining wide appeal for many uses, from healthcare and medicine to energy and environmental applications. Phosphorus-Based Polymers is the first book dedicated to this topic and provides a comprehensive overview of the different polymers and their uses.
The first part of the book covers the synthesis and polymerisation of different phosphorus containing systems including phosphorus containing (meth)acrylate, (meth)acrylamide and viny or allyl monomers, as well as vinyl phosphonic acid, 2-methacryloyloxyethyl phosphorylcholine, poly(phosphoesters)and polyphosphazenes. The second part of the book contains specific chapters detailing different applications such as biomedical applications in dental materials, tissue engineering and drug delivery, metal complexation for anti-corrosion and wastewater purification materials, fire retardant additives and fuel cell membranes.
Written by expert researchers in the chemistry of phosphorus-containing polymers, this book is suitable for academic and industrial researchers interested in polymer and materials synthesis as well as their applications.
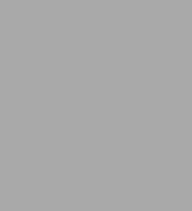
Phosphorus-Based Polymers: From Synthesis to Applications
318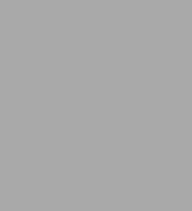
Phosphorus-Based Polymers: From Synthesis to Applications
318eBook
Available on Compatible NOOK devices, the free NOOK App and in My Digital Library.
Related collections and offers
Overview
Phosphorus-containing (co)polymers are gaining wide appeal for many uses, from healthcare and medicine to energy and environmental applications. Phosphorus-Based Polymers is the first book dedicated to this topic and provides a comprehensive overview of the different polymers and their uses.
The first part of the book covers the synthesis and polymerisation of different phosphorus containing systems including phosphorus containing (meth)acrylate, (meth)acrylamide and viny or allyl monomers, as well as vinyl phosphonic acid, 2-methacryloyloxyethyl phosphorylcholine, poly(phosphoesters)and polyphosphazenes. The second part of the book contains specific chapters detailing different applications such as biomedical applications in dental materials, tissue engineering and drug delivery, metal complexation for anti-corrosion and wastewater purification materials, fire retardant additives and fuel cell membranes.
Written by expert researchers in the chemistry of phosphorus-containing polymers, this book is suitable for academic and industrial researchers interested in polymer and materials synthesis as well as their applications.
Product Details
ISBN-13: | 9781782621515 |
---|---|
Publisher: | RSC |
Publication date: | 05/07/2014 |
Series: | Polymer Chemistry Series , #11 |
Sold by: | Barnes & Noble |
Format: | eBook |
Pages: | 318 |
File size: | 7 MB |
About the Author
Sophie Monge is assistant professor in Macromolecular Chemistry at the University of Montpellier 2, in the ICGM laboratory. She is an expert in controlled radical polymerization, especially atom transfer radical polymerization (ATRP) and reversible addition-fragmentation transfer polymerization (RAFT). This includes the synthesis of various molecules necessary for the polymerization reactions such as ligands, initiators, chain transfer agents, etc...and their characterization. An important field of her research deals with the synthesis and polymerization of phosphorus-containing polymers for various applications, in particular the biomedical field, and the complexation with metals.
Ghislain David is assistant professor in Macromolecular Chemistry at the National School of Chemistry of Montpellier, in the ICGM laboratory. His main research projects are in the field of phosphorus-containing polymers obtained either from synthesis and radical polymerizations of phosphorus-containing monomers or from post-modification of pre-made polymers. Thus he is an expert in organo-phosphorus chemistry aiming at synthesizing of new monomers and polymers having phosphonate moieties. His research interests concern the developments of such polymers for a large field of applications: membranes for fuel cells, anti-corrosive coatings and flame retardants.
Read an Excerpt
Phosphorus-Based Polymers
From Synthesis to Applications
By Sophie Monge, Ghislain David
The Royal Society of Chemistry
Copyright © 2014 The Royal Society of ChemistryAll rights reserved.
ISBN: 978-1-84973-646-6
CHAPTER 1
Polymerization of Phosphorus-Containing (Meth)acrylate Monomers
SOPHIE MONGE, BENJAMIN CANNICCIONI, GHISLAIN DAVID AND JEAN-JACQUES ROBIN
Institut Charles Gerhardt de Montpellier, UMR5253 CNRS-UM2-ENSCM-UM1, Equipe Ingénierie et Architectures Macromoléculaires, Université Montpellier II, cc1702, Place Eugène Bataillon, 34095, Montpellier, France
1.1 Introduction
In recent years, phosphorus-based polymers have been widely studied as they exhibit very unusual and interesting properties. Whereas the ester forms are the most available compounds, monoacids and diacids can be easily obtained under mild conditions with the use of bromotrimethylsilane, opening the way to a wide range of polymers showing different properties. The latter can be explained in part by the ionization potential of phosphonic acids, which is intermediate between that of sulfonic and carboxylic acids due to their intermediate pKa.
Phosphorus-containing materials can be employed for a wide range of technological applications. For instance, they are extensively used in industry, notably to bind metals. Indeed, phosphorus-based materials show interesting complexing properties and are used as dispersants, corrosion inhibiting agents, or for preventing deposit formation. They are also involved in flame retardancy, where phosphorus is known to be particularly useful. An important industrial application deals with their use in the biomedical field, as they are biodegradable, blood compatible, show reduced protein adsorption, and lead to strong interactions with dentin, enamel, or bones. As a consequence, various syntheses of monomers and polymers are carried out following different procedures: (i) introduction of the phosphorinated moieties onto polymers by (co)polymerization of monomers bearing the phosphorus atom or (ii) grafting of phosphorus-based groups onto the polymer.
In this contribution, we will focus on the polymerization of phosphorus-based (meth)acrylates. Different kinds of monomers will be considered, as a function of the targeted applications. The latter will be more thoroughly discussed in the second part of this book (Chapters 8–13). The resulting phosphorus-based poly(meth)acrylates are mainly used for anticorrosion, flame retardancy, tissue engineering, and dental applications. Concerning anticorrosion, polymers have been involved in corrosion protective coatings which require maintenance of adhesion under environmental exposure. Adhesion between galvanized steel plates and the polymer depends on the chemical structures of both substrate and coating and reduction of adhesion causes water penetration at the coating/metal interface, leading to a significant reduction of adhesion. Incorporation of phosphonic groups, known as adhesion promoters, into polymer structures allows improvement of the adhesion properties of polymers on metallic surfaces. Furthermore, research has also been carried out on the development of new halogen-free flame retardants. In this context, phosphorus is known to be efficient with or without other elements like nitrogen or sulfur. Concerning tissue engineering, polymeric materials, especially phosphonated polymers, have already proved to be of great interest. Tissue engineering typically involves the seeding of biodegradable polymeric scaffolds with differentiated or pluripotent cells in vitro, followed by implantation of the whole cell–scaffold system into the region of tissue loss or damage. It has been shown that protein interactions were favored when phosphorus-grafted polymeric surfaces were used. Finally, among all self-etching adhesive systems developed in dentistry for bonding of resin composite to enamel or dentin, primers containing phosphonated or phosphonic acid groups have been quite widely considered. Indeed, such derivatives are potentially interesting as the incorporation of a phosphonic function would result in an increase of the biocompatibility and in the adhesion due to chelation with calcium ions at the tooth surface because of complex formation with calcium in hydroxyapatite. This mineral is partially dissociated by phosphonic acid to give brushite acting as a macromolecular crosslinker and the bond strength depends on the alkyl chain length of the monomer. For this last application, bis(meth)acrylate monomers are also considered. Finally, special attention will be paid to the controlled radical polymerization of dimethyl [(methacryloyloxy)methyl]phosphonate, very recently reported in the literature, demonstrating that it is possible to prepare well-controlled architectures involving phosphorus-based monomers.
1.2 Synthesis by Free or Controlled Radical Polymerization of Phosphorus-Based Poly(meth)acrylates as Adhesion Promoters
Free-radical graft copolymerization of phosphonated (meth)acrylates onto polymers is carried out with dimethyl [2-(meth)acryloxyethyl]phosphonate (DAP) or corresponding mono- or diacid derivatives (DAP monoacid or DAP diacid) (Scheme 1.1). These monomers are obtained using a simple synthetic method starting from commercial compounds. DAP is obtained by an esterification reaction involving acryloyl chloride and dimethyl (2-hydroxyethyl)phosphonate in dichloromethane in the presence of triethylamine. The synthesis of phosphonic acid monomer derivatives (DAP monoacid and DAP diacid) is achieved in two steps: (i) silylation of a dimethyl phosphonate function by bromotrimethylsilane, followed by (ii) hydrolysis with an excess of methyl alcohol. Using an equivalent amount of brominated silane and DAP leads to a mixture of three functional phosphonated groups (notably with DAP monoacid), whereas an excess of bromotrimethylsilane quantitatively converts phosphonated moieties into phosphonic diacid groups (DAP diacid). Then, ozone-oxidized poly(vinylidene difluoride) (PVDF) is grafted with phosphonated acrylate monomers and acrylic acid by two methods: solution or bulk polymerization. Polymerization in solution is achieved using dimethylformamide (DMF) as solvent at 90 °C for 24 h, or deionized water at 60 °C for 12 h. For bulk polymerization, the reaction is carried out at 130 °C in an air atmosphere for the prescribed time. Then, the graft copolymer is dissolved in DMF and the homopolymer is removed by precipitation in ethyl alcohol. Adhesion of the graft copolymers was subsequently studied. The presence of phosphonic acid groups leads to a significant improvement in the adhesive bond to metal compared to both dialkyl phosphonate and carboxylic acid groups. The same kinds of experiment were carried out with low-density polyethylene powders using phosphonated methacrylates by grafting in the presence of free-radical initiators or thermally induced graft copolymerization onto ozone-pretreated low-density polyethylene.
Poly(MMA)-b-poly(monophosphonic methacrylate) (MMA=methyl methacrylate) diblock copolymers are also prepared by atom transfer radical polymerization (ATRP) and used as additives in PVDF coatings to protect steel against corrosion. ATRP of dimethyl [(methacryloyloxy)methyl]phosphonate (MAPC1), prepared by the Pudovic reaction, was investigated in toluene, in the presence of methyl 2-bromoisobutyrate as the initiator, and using different metal and ligand systems. Polymerization proceeded with very low monomer conversion, which was attributed to the ability of phosphorus to complex the copper ions, removing copper ions from the original ligand stopping the MAPC1 polymerization. As a result, another strategy was chosen for an efficient synthesis of poly(MMA)-b-poly(phosphonate acrylate) diblock copolymer (without phosphonated-based monomer), which was efficiently obtained by a four-step reaction, with first the synthesis of poly(MMA)-b-poly(tert-butyl acrylate) diblock copolymer by ATRP. Then the tert-butyl groups were removed and phosphonate functions were incorporated by esterification. This new diblock copolymer was used as an additive for an anticorrosive coating, but no improvement (using the salt spray test technique) was observed compared with the statistical copolymer with the same acid content.
Blends of phosphorus-containing copolymers with PVDF powders were also investigated to enhance adhesive and anticorrosive properties of fluoro polymer coatings. Statistical copolymers were first prepared copolymerizing MMA and dimethyl [(2-methacryloyloxy) ethyl]phosphonate, [(2-methacryloyloxy)ethyl]phosphonic diacid, or methyl [(2-methacryloyloxy)ethyl]phosphonic hemiacid. Concerning the synthesis of the monomers involved, dimethyl (2-hydroxyethyl)phosphonate reacted with methacryloyl chloride in the presence of triethylamine in dichloromethane. The resulting dimethyl [(2-methacryloyloxy)ethyl]phosphonate was isolated by distillation under vacuum (2×10-3 mbar) at 85 °C. The acid derivatives were synthesized by methanolysis using bromotrimethylsilane and methanol. Copolymerizations were then carried out at 70 °C either in THF or DMF in the presence of AIBN. The resulting copolymers were introduced into PVDF as adhesion promoters and anticorrosion inhibitors. Good dry and wet adhesion properties onto galvanized steel plates were obtained with blends containing a high content of phosphonic acid groups. A salt spray exposure test also showed that the phosphonic acid groups prevented the spread of corrosion at the PVDF coating/galvanized steel interface. MAPC1, with only one methylene spacer, was copolymerized with MMA. Radical copolymerizations of both MAPC1 and its acidic form, MAPC1(OH), with MMA were carried out in acetonitrile at 80 °C, initiated by AIBN. When MAPC1 was used, the phosphonated ester moieties were then hydrolyzed. When blended with PVDF and coated onto a galvanized steel plate, the copolymer led to very good adhesion in both wet and dry states and provided good anticorrosion properties.
The reversible addition–fragmentation chain transfer (RAFT) polymerization of vinylidene chloride, methyl acrylate, and a phosphonated monomer named MAUPHOS [CH2=C(CH3)C(O)O[(CH2)2]NHC(O)O(CH2])2 P(O)(OCH3)2] has been achieved. The phosphonated methacrylate was synthesized by reacting isocyanoethyl methacrylate and the appropriate phosphonated alcohol. Unlike usual methods (esterification and transesterification), this synthesis was quantitative and easy to realize. RAFT polymerization was carried out at 70 °C in benzene under pressure using 1-(ethoxycarbonyl)ethyl dithiobenzoate as a chain transfer agent in an autoclave because of the low boiling point of the vinylidene chloride, and yielded a gradient terpolymer which was then hydrolyzed. The latter was used as polymeric additive in coating formulations based on a poly(vinylidene chloride-co-methyl acrylate) copolymer matrix. Different formulations were spun cast on stainless steel surfaces and the coatings were analyzed by electron microscopy coupled with X-ray analyses. Phosphonic acid groups induced preferential organization of the coating as the additive segregated and migrated toward the metal interface. As a consequence, the matrix at the air interface acted as a barrier to gas while the additive ensured adhesion at the polymer/metal interface. Another terpolymer was also evaluated, combining a methacrylic phosphonated monomer copolymerized with MMA and butyl methacrylate. The phosphonated monomer was obtained from diethyl 1,2-epoxyprop-2-ylphosphonate and methacrylic acid in the presence of tetrabutylammonium chloride in toluene at 80 °C, with 76% yield. Then, radical terpolymerization took place with AIBN in acetonitrile at 80 °C. In the case of the resulting terpolymer, high resistance towards corrosion was observed as no corrosion occurred even after 1100 h of the salt spray test. Innovative multifunctional phosphonated monomers were incorporated into photopolymerizable mixtures of (meth)acrylates and showed, after UV hardening, good adhesion onto steel and excellent resistance to corrosion under salt spray. The phosphonated monomers were propyl N,N-tetramethylbis(phosphonate)-2-hydroxylbismethyleneamine methyl methacrylate (for n=3) (MAC3NP2) and 2-[2,2-bis(diisopropoxyphosphoryl)ethoxy]methyl methacrylate (MAC3P2) (see Scheme 1.1). The amine-containing methacrylate MAC3NP2 was synthesized via a three-step procedure, where the corresponding aminodiphosphonate hydroxy compound was prepared by the Kabachnick–Field process. It was subsequently esterified and the obtained aminobisphosphoryl methacrylate monomer was finally hydrolyzed, resulting in the bisphosphoryl methacrylate monomer in 60% yield. The synthesis of MAC3P2 was achieved with a similar synthetic strategy to the one used for the MAC3NP2 monomer, leading to MAC3P2 in a yield of 85%.
1.3 Synthesis by Radical Polymerization of Phosphorus-Based Poly(meth)acrylates for Flame Retardancy
One of the main drawbacks of the usual polymeric materials is their flammability and the emission of very hazardous gases and smoke during combustion. Only a few polymers are able to self-extinguish themselves. Among all possibilities, phosphorus-based materials have proved to be efficient in this respect. However, the design of new phosphonated halogen-free retardant additives is not an easy task. No universal compound exists and each additive is associated with one polymer type. This observation comes from the degradation mechanisms that differ from one polymer to another. Indeed, some polymers are able to self-generate chars when burning while others do not have this property. In the case of char formation, some phosphorus- based compounds can be easily integrated in this char and have a positive action in the formation of this porous and protective foamed material. Results reported in the literature have dealt with the copolymerization of (meth)acrylic monomers bearing phosphonate or phosphate groups, namely diethyl [(methacryloyloxy)methyl]phosphonate (DEMMP), diethyl [(acryloyloxy)methyl]phosphonate (DEAMP), diethyl (methacryloyloxy)ethyl phosphate (DEMEP), and diethyl (acryloyloxy)ethyl phosphate (DEAEP) (Scheme 1.2).
These studies clearly showed that the chemical structure of the phosphorus atom plays a major role in the flame retardancy, with phosphonate groups proving to be more active than the corresponding phosphate groups. Furthermore, phosphonated groups linked to the polymer backbone and obtained by copolymerization had a low effect on mechanical and physical properties in comparison with phosphonated compounds added to polystyrene (PS), where a decrease of these properties was obtained. The additives acted in the vapor phase, whereas phosphonated copolymers were active both in the vapor and condensed phases.
Phosphorus-based (meth)acrylates were also combined with poly(MMA). In this work, DEMMP was synthesized by the condensation of methacryloyl chloride with diethyl (hydroxymethyl)phosphonate in the presence of triethylamine in anhydrous dichloromethane under argon at room temperature. Plaques of MMA and DEMMP copolymers, containing different molar ratios of DEMMP, were prepared by heating nitrogen-bubbled mixtures of MMA, DEMMP, and AIBN in an oven. Copolymers of MMA and DEMMP were also prepared in solution at 60 °C using AIBN as initiator. Copolymers made in solution were then pressed into plaques. It was shown that DEMMP acted in the condensed phase but also in the vapor phase, and char formation as well as the autoignition temperature were greatly increased for 10 mol% content. Some mechanisms operating during the formation of char were proposed in a complementary study. The well-known mechanism of depolymerization of PMMA was disturbed in the presence of DEMMP. Free additives containing PMMA afforded lower flame retardancy properties than those of additives incorporated in PMMA chains. Furthermore, the authors showed that phosphonate monomers were more efficacious than the corresponding phosphate ones. More recently, the same authors studied in detail the mechanisms occurring during thermal degradation of PMMA and showed the effect of the chemical structure of the phosphonated comonomer (acrylate or methacrylate) on the PMMA backbone decomposition.
Thermosetting resins like UV curable resins have also been studied and the effect of phosphonated monomers was evaluated. Monomers bearing two phosphonic acid groups (Scheme 1.2, right) showed a good effect on the flame retardancy. Phosphorus-containing acrylate monomers were synthesized from the reaction of ethyl (chloromethyl)acrylate and tert-butyl (bromomethyl)acrylate with triethyl phosphite. The selective hydrolysis of the ethyl ester monomer with bromotrimethylsilane gave a phosphonic acid monomer. The attempted bulk polymerizations of the monomers at 57–60 °C with AIBN were unsuccessful, but bulk copolymerization with methyl methacrylate at 60 °C with AIBN was performed. Additionally, α-(chloromethyl)acryloyl chloride was reacted with diethyl (hydroxymethyl)phosphonate to obtain another monomer. This monomer was hydrolyzed, homopolymerized, and copolymerized with MMA. For instance, the photopolymerization of this monomer gave 90% conversion in 60 s. More generally, the relative reactivities of the synthesized monomers in photopolymerizations were determined and compared with those of the other phosphorus-containing acrylate monomers. Changing the monomer structure allowed control of the polymerization reactivity so that new phosphorus-containing polymers with desirable properties could be obtained.
(Continues...)
Excerpted from Phosphorus-Based Polymers by Sophie Monge, Ghislain David. Copyright © 2014 The Royal Society of Chemistry. Excerpted by permission of The Royal Society of Chemistry.
All rights reserved. No part of this excerpt may be reproduced or reprinted without permission in writing from the publisher.
Excerpts are provided by Dial-A-Book Inc. solely for the personal use of visitors to this web site.
Table of Contents
Polymerization of Phosphorus-Containing (Meth)acrylate Monomers;
Polymerization of Phosphorus-Containing (Meth)acrylamide Monomers;
Phosphorus-Containing Both Vinyl or Allyl Monomers;
Synthesis and Polymerization of Vinyl Phosphonic Acid;
2-Methacryloyloxyethyl PhosphorylCholine (MPC) Polymers;
Polyphosphoesters;
Polyphosphazenes High Polymers;
Phosphorus-Based Monomers Used for Dental Application;
Biomedical Applications of Phosphorous-Containing Polymers;
Complexation with Metals: Anti-Corrosion;
The Use of Phosphorus-Containing Polymers for the Removal of Metal Ions from Wastewaters;
Flame Retardancy of Phosphorus-Containing Polymers;
Proton Conducting Phosphonated Polymers and Membranes for Fuel Cells;