Quantitative Textural Measurements in Igneous and Metamorphic Petrology available in Hardcover, Paperback
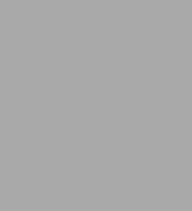
Quantitative Textural Measurements in Igneous and Metamorphic Petrology
- ISBN-10:
- 052113515X
- ISBN-13:
- 9780521135153
- Pub. Date:
- 03/11/2010
- Publisher:
- Cambridge University Press
- ISBN-10:
- 052113515X
- ISBN-13:
- 9780521135153
- Pub. Date:
- 03/11/2010
- Publisher:
- Cambridge University Press
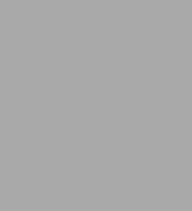
Quantitative Textural Measurements in Igneous and Metamorphic Petrology
Buy New
$63.99Overview
Product Details
ISBN-13: | 9780521135153 |
---|---|
Publisher: | Cambridge University Press |
Publication date: | 03/11/2010 |
Edition description: | Reissue |
Pages: | 276 |
Product dimensions: | 6.70(w) x 9.60(h) x 0.70(d) |
About the Author
Michael Higgins is Professeur for Sciences de la Terre at the Université du Québec ... Chicoutimi, Canada. He is a member of the Geological Association of Canada.
Read an Excerpt
Cambridge University Press
0521847826 - Quantitative Textural Measurements in Igneous and Metamorphic Petrology - by Michael Denis Higgins
Excerpt
1
Introduction
I do not know what I may appear to the world, but to myself I seem to have been only like a boy playing on the sea-shore, and diverting myself in now and then finding a smoother pebble or a prettier shell than ordinary, whilst the great ocean of truth lay all undiscovered before me.
Sir Isaac Newton
Newton’s ‘Ocean of Truth’ seems to me more like a landscape: the plains are densely populated with information and most researchers work there. It is not easy to get a perspective on such a mass of information without climbing the surrounding hills. Valleys in the mountains may be difficult to find, and sparsely populated, but some lead to new basins of information ready to be explored. Other valleys may be so deep that we can glimpse what they contain, but cannot explore them closely, or even at all. This book is a guide to a country set in that landscape. Like real countries, its name varies according to who you ask, and the borders do not always follow geographic features. And like most travel writers, my description of the landscape is coloured by where I come from and what I have done.
1.1 Petrological methods
In petrology1 we generally examinethe results of natural experiments and have to tackle the inverse problem of what happened to what starting material to produce the rock that we observe. This problem is complex and does not usually have a unique solution: we must decide what the most likely starting material was and what the dominant processes were. The general approach is to choose a number of parameters and quantify them in the final rock. A starting material is proposed and various processes applied to this material. The results of this modelling are then compared to the rock in question.
All rocks comprise an assemblage of mineral grains of different sizes, orientations and shapes, which may be observed in many different ways. Early petrological studies of rocks used observations in the field and with a polarising microscope. The value of chemical analyses was appreciated, but the effort involved to analyse a small number of samples was considerable. The development of instrumental methods of chemical and isotopic analysis changed this and now such methods have become very important. In fact, many geologists view igneous and metamorphic petrology as simply applied mineral, chemical and isotopic analysis. This is a rather narrow view and in this book I hope to show that quantitative analysis of textures can also help unravel the evolution of materials. In a way, textural methods are more direct than geochemical or isotopic methods as the most important processes involve changes in the size, shape, position and orientation of the crystals. However, it is better to view all these methods as complementary.
This book is aimed at researchers in igneous and metamorphic petrology at all levels. Some workers may not be aware of the potential of textural studies to solve problems that they have previously attacked only with chemical or isotopic methods. Others may have started textural studies, but are unaware of what has been done in other subfields of petrology.
An ideal petrological study would combine relevant theory, appropriate analytical methods and application to real rocks. However, petrologists are human and in many cases one aspect is developed to the detriment of the other: the siren calls of theory and methods seem to be heard more often than application to rocks. I hope that readers will be able to combine both high-quality measurements with well-grounded theory to advance our understanding of the origin of rocks.
1.2 Qualitative versus quantitative data
Although the patterns and structures of rocks have been observed and recorded since early times, it was only in the nineteenth century that the development of the polarising microscope enabled a vast increase in the detail and number of petrological studies. At that time such observations were mostly qualitative – such as average grain-sizes, grain relationships, grain boundary shapes and orientation fabrics. While qualitative data have their uses, they cannot constrain physical models of processes in the same way that quantitative data can (for a history of quantification in geology see the review of Merriam, 2004). Models can be developed that make quantitative predications which can only be verified if accurate quantitative observations of rocks are available. All too often authors have developed elaborate mathematical models of geological processes, only to remark at the end of the article that their results qualitatively resemble actual rocks. What are clearly needed are quantitative measurements of rock textures that can be compared to theoretical predictions.
The division between qualitative and quantitative data is somewhat artificial and relates to aspects of data quality. In quantitative studies data quality refers largely to the accuracy of the measurements. If data are not accurate then they may be no more useful than qualitative data and indeed may be detrimental. In good geochemical studies accuracy is proved by analysis of geochemical reference materials. Although there are a few such materials available for textural analysis they are of limited application and are rarely used.
1.3 What do I mean by texture?
The texture of a rock is considered here to be the geometric arrangement of grains, crystals, bubbles and glass in a rock. I use the term rock here in its most general sense: for both lithified and loose materials. Materials scientists and geophysicists also use the term polycrystal to describe the same sorts of materials. Although the internal structure of grains and crystals is very important, and may help our interpretations, it is not developed here. The term texture as used here includes orientation of crystals, which is sometimes separately referred to as fabric. In other fields the terms fabric and texture are used in the opposite way. In materials science the term microstructure covers the same field (Brandon & Kaplan, 1999) and this is favoured by some geologists (Vernon, 2004). However, the name of the subject is not really important, as compared to its content.
A number of textural components can be, and are commonly quantified:
- Size of grains, crystals and bubbles.
- Shape and boundary convolutions of grains and crystals.
- Orientation of grain and crystal shapes and crystal lattices.
- Position and connectedness of crystals.
- The relationships between different phases.
Other textural components, such as enclosure relationships, are important but are not usually quantified. They can be useful as earlier textures may be preserved within oikocrysts or other structures (e.g. Higgins, 1998).
The precision and accuracy of textural parameters determined from rocks is very variable, but does not correlate with the relevance of these parameters to current problems in petrology. However, if a parameter can be readily and accurately measured, then it should be reported or made available in an electronic archive, even if its relevance is not apparent when the study is done: we have little idea what will be needed for future studies.
Quantitative textural analysis of rocks must start with measurement of textural parameters in rocks. Modelling of the evolution of these parameters is generally done by taking each parameter individually. For example crystal size is generally modelled independently from other textural parameters (e.g. the classic study of Cashman & Marsh, 1988). This probably reflects on the youthful nature of the field, as compared to geochemical or isotopic studies. However, there have been some attempts to model the whole 3-D texture of a rock mathematically.
1.4 Information density and data sources
One of the problems inherent in a book such as this is the huge variation in the number of studies in different branches of the subject. I call this the information density. For example, there are hundreds, or maybe thousands, of studies of the orientation of crystals in metamorphic rocks, yet probably less than ten in igneous rocks. This means that in some fields I have just summarised the field, referred to a specialised text or discussed a few key studies, whereas in others I mention all work in the subject. A subjective assessment of information density is shown in Table 1.1.
One of the most important roles of this book is to broaden the application of methods across the subject, and hence to even out these variations in information density.
Scientific data are circulated in many forms: some are rigorously refereed and easily accessible, whereas others do not meet these criteria. In this work I have not referenced or used short conference abstracts as they are not really refereed and rarely contain enough information to be useful. Often they seem to be just marking out territory. Many M.Sc. and Ph.D. theses do contain useful data and methods, but they are not refereed to the same standards as most journal
Table 1.1 Estimates of information density in quantitative textural studies: O = little work, OOO = many studies.
Field of study | Igneous petrology | Metamorphic petrology |
Crystal and pore size | OO | O |
Crystal shape | O | O |
Crystal orientation | O | OOO |
Crystal position | O | O |
papers. In addition, theses are not always easy to come by and their existence and content tends to be passed on by word-of-mouth. Hence, I have generally omitted theses: high-quality studies will generally be published anyway by the student or the supervisor.
1.5 Structure of this book
Although this book covers a wide range of quantitative textural approaches to petrological problems many share similar analytical methods. Hence, I start in Chapter 2 with such generally applicable methods. I will then divide the subject on the basis of the parameters measured: size, shape, orientation and position. In Chapters 3 to 6 I will briefly review enough theory so that the nature of the problems can be understood. I then continue with a survey of the analytical methods, where they differ from the more generally applicable methods discussed in Chapter 2. The data produced by some analytical methods require significant treatment to extract meaningful textural parameters. I will present some applications that are typical of this subject, innovative, or which show the way that the subject may develop in the future. If I make too many references to my work and that of my students, then it is because I am familiar with the data, and can recalculate them to compare them with the data of others. Finally, I finish with Chapter 7 on all aspects of the textures of pores.
1.6 Software applications for quantitative textural studies
The analytical methods described in this book use a variety of different software applications. Many researchers are unwilling or unable to invest the time and money needed to acquire new software and the skills to use it. This is particularly evident if the software is not free-standing, that is, it must be used with other applications. These factors result in a tremendous range in the application of new methods that is not entirely dependent on their overall scientific usefulness. A caveat for researchers is that if you want your method to be widely employed, then you must make it easy and cheap to use. Software types and mathematical procedures are presented below in decreasing order of likelihood of use.
- General-purpose image processing and spreadsheet programs (e.g. Adobe Photoshop and Microsoft Excel). These are available for a number of operating systems (Windows, Mac, Linux, etc.). A lot of data reduction can be done with such programs, but they are commonly oriented towards business applications and hence may involve rather complex manipulations to do simple calculations. Sometimes it is easier to augment these programs by writing a small program to do one step in the processing or to convert data formats.
- Special programs: freeware, shareware and commercial. Some programs are readily available (e.g. CSDCorrections, NIHImage) while others can be very expensive. Most freeware is not available in all operating systems. However, Java programs (e.g. ImageJ) can be used on most operating systems. There are a number of large and comprehensive statistical packages that can be used to manipulate data (SAS, SPSS).
- Macros (procedures) for high-level languages, such as Mathematica, Maple, Matlab (Middleton, 2000), ImageJ and IDL. Such languages are very powerful but are not so readily available. They have a steep learning curve for beginners. Many academic researchers may not realise that they already have access to these packages, sometimes via engineering departments. Many compiled IDL programs can be run, but not modified, with the aid of a free program called a ‘Virtual Machine’.
- Uncompiled program code in common languages such as FORTRAN, C, Pascal or Basic. Such code must be compiled for the operating systems that will be used. Many compilers are commercial and can be expensive. Minor code modifications are commonly needed and necessitate some knowledge of programming or a helpful assistant.
- Mathematical equations and algorithms. Some equations are easily applied but others necessitate that software must be written before the method can be applied.
- ‘Home-made’ software. Software development with ‘visual’ compilers like Visual Basic, Visual C or Delphi (= visual Pascal) is relatively easy for simple applications, but difficult to perfect. If you have no programming experience you should expect to spend a week before you can make a simple program. Visual Basic is slow and should not be used for calculation intensive applications. Visual C or Delphi are not much more difficult to learn and produce a faster program.
All methods should ideally be tested with standard materials or synthetic data derived using independent methods, but this is not always available. Software ‘bugs’ and operating system incompatibilities are inevitable – producers of scientific software cannot usually test their programs on all systems and generally welcome constructive comments. They are generally geologists and not programmers. They do not like to hear ‘your program never worked so I abandoned it’.
Software mentioned in this book is described in the Appendix and also on a website, which will be updated regularly (http://geologie.uqac.ca/%7Emhiggins/CSD.html).
Notes
1. In this book I will address igneous and metamorphic petrology. Quantification of textures is an important part of sedimentary petrology, particularly grain-size analysis of unconsolidated sediments, but the subject is too vast and distant from the petrology of crystalline rocks to be bridged by one author in one book.
2
General analytical methods
2.1 Introduction
2.1.1 Observations in three and two dimensions
Many different analytical methods have been applied to quantifying the textures of rocks, but all start with observations of rocks either in three or two dimensions. In this chapter I will start with general analytical methods that apply to the determination of many different textural parameters. Methods specific to a particular textural parameter will be discussed later in the relevant chapters. It should be remembered that different methods can be combined within a study. This can validate data acquired by innovative methods and also extend the range of grain sizes that can be quantified (Figure 2.1). Some methods that I will describe are used widely (e.g. transmitted light microscopy) whereas others are discussed here because I think that they have potential for future textural studies (e.g. Nomarski imaging). Researchers do not always choose their analytical method in the most logical way: here I want to survey the field to show what others have done. However, researchers, especially students, should never forget that a lot can be done with little equipment – my first crystal size distribution study used a microscope, camera, ruler and protractor (Higgins, 1991).
The texture of many different objects or parts of objects in a rock can be described: it is important to specify at the start what you want to examine. For instance, if a crystal is fractured then the size of the fragments or the size of the original crystal can be measured. If grains are altered at their rims then the original size of the grain or the present size of the grain can be measured.
The texture of rocks is a three-dimensional (3-D) property, hence it is most directly studied by analytical methods that view blocks of rock. However, such methods are not always applicable: samples, and grains, may be too large or small for 3-D methods; the equipment may not be available or too expensive
Image not available in HTML version |
Figure 2.1 Quantitative textural methods that can be used at different scales of interest. Electronic methods include scanning electron microscopy (SEM), electron microprobe (EMP), transmission electron microscopy (TEM) and cathodoluminescence. The scale is the linear dimension of the sample – the edge of the surface or block. Maximum grain or crystal sizes that can be measured successfully are typically about one tenth of these values. Not all methods can give all textural parameters: for example, sample solution cannot give information on crystal position or orientation. Even within the scale range of a single method it may be necessary to gather the information at two different magnifications.
for the study; and many 3-D methods do not distinguish clearly touching grains of the same phase. Hence, it is commonly necessary to study sections through a rock, and extrapolate these data to three-dimensional textural parameters. The branch of mathematics that deals with this problem is known as stereology (Underwood, 1970). Detailed stereological methods will be discussed in the relevant chapters. However, here I will mention a number of ‘global parameters’, which describe uniquely various geometrical properties of a solid and can be determined from examination of a section. Finally, I will briefly describe some of the more general methods used to summarise the distribution of textural parameters, whether determined by 2-D or 3-D analytical methods.
2.1.2 Grain size limits
The size limits and resolution of different analytical methods must be respected (Figure 2.1). The resolution of many analytical methods is clear – for two-dimensional images it is commonly the size of the pixels (picture elements – dots or groups of different coloured dots on the screen). However, other factors may reduce the resolution, such as the lenses or physical limits. The minimum size of grain or crystal that can be measured accurately must be established from this value. For size measurements a typical limit might be ten times the resolution: for example, each measured crystal must have a length of at least 10 pixels. If a method uses discrete intervals, then there must also be sufficient grains or crystals in an interval such that the value is statistically meaningful. For example, if a size interval contains only one crystal then that value has very little meaning. These factors generally limit the maximum size of grains or crystals that can be quantified to about one tenth of the size of the image.
2.1.3 Edge effects
Almost all analytical methods, both 3-D and 2-D, must deal with the problem of edge effects of samples. Boundaries of a sample commonly intersect some crystals, which must be dealt with in a consistent manner. If the sample contains many crystals then edge effects can be ignored. For smaller samples two simple solutions are possible. The area or volume of measurement need not have a simple shape and can be a complex envelope that only includes completely visible crystals (Figure 2.2a). The area or volume of measurement can be a simple rectangle or parallelepiped that is drawn inside the actual limits of the sample so that partially visible crystals are excluded (Figure 2.2b). Then only those crystals that touch two sides of the square, or three edges of the parallelepiped, are included.
2.1.4 Textural development sequences
When a rock is sampled all that can be generally observed is the final product – the path taken to achieve that product is not always clear, but may sometimes
Image not available in HTML version |
Figure 2.2 Two solutions to the problem of edge effects in textural measure-ments, shown here for a section. (a) Crystals that are not completely visible in the field of view (open outlines) are excluded and an irregular envelope passing midway between the crystal edges (dotted line) encloses the crystals to be measured (grey outlines). The area of the envelope is the area measured. (b) A rectangle is drawn around all crystals that are completely visible. Crystals are counted that fall within the rectangle or touch two of the sides. Those that touch the other two sides (dashed) are excluded as well as those completely outside the rectangle.
be revealed by carefully examining other, less developed samples, or by locating early textures frozen in by other processes. Such sequences of textural development can help enormously in understanding petrogenetic processes. For instance a series of lavas or samples taken from a lava lake may show how magmas crystallise (e.g. Cashman & Marsh, 1988). First-formed plutonic textures may be seen in oikocrysts and early metamorphic textures may be preserved in porphyroblasts (e.g. Higgins, 1998). Hence, the methods described below should be applied not only to the average rock, but also to special sectors of a sample that can show evidence of earlier textures.
2.2 Complete three-dimensional analytical methods
Three-dimensional analytical methods that conserve the size, shape, orientation and position of the crystals will be discussed first. Some of these methods conserve the sample (e.g. X-ray tomography) whereas others are destructive (e.g. serial sectioning).
2.2.1 Serial sectioning
The complete texture of a sample can be established from serial sectioning (Bryon et al., 1995). Here, a surface or thin section is cut and recorded as a photograph or digital image. The surface is then ground away and a new
© Cambridge University Press