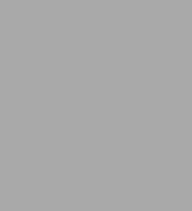
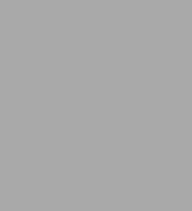
eBook
Available on Compatible NOOK devices, the free NOOK App and in My Digital Library.
Related collections and offers
Overview
Product Details
ISBN-13: | 9780486174532 |
---|---|
Publisher: | Dover Publications |
Publication date: | 10/09/2012 |
Series: | Dover Books on Physics |
Sold by: | Barnes & Noble |
Format: | eBook |
Pages: | 160 |
File size: | 3 MB |
Read an Excerpt
READABLE RELATIVITY
By CLEMENT V. DURELL
Dover Publications, Inc.
Copyright © 2014 Dover Publications, Inc.All rights reserved.
ISBN: 978-0-486-17453-2
CHAPTER 1
THE PROGRESS OF SCIENCE
"We know very little and yet it is astonishing that we know so much, and still more astonishing that so little knowledge can give us so much power."—BERTRAND RUSSELL, The ABC of Relativity.
Our Outdoor World.
Every one nowadays has heard of Einstein and Relativity. The man in the street, however, still treats the subject more as a fairy tale or a mathematical jest than as a contribution to scientific knowledge and method which is as momentous (if it stands the test of continued scrutiny) as any that has ever been made. Relativity is a branch of physics, not of pure mathematics. Its conclusions could not, of course, have been obtained without the aid of pure mathematical reasoning of a difficult and abstruse nature, but the mathematical side is incidental: mathematics has merely supplied the machinery for working on the material available and the language for describing the result. The material itself is the product of experiment, observation, and measurement. The whole aim of Natural Science is to examine what is happening in our outdoor world, the Universe in which we live, and to construct the simplest set of suppositions which will cover all the observed facts.
The sole question, therefore, that arises is this: Does Einstein's theory of Relativity give a more harmonious and adequate picture of what is observed to be happening in the Universe than any other alternative scientific theory dealing with the same data, or not?
Many articles in newspapers and magazines seem to suggest that the Relativity creed contains such fantastic propositions that, however much they may appeal to philosophers, they cannot be taken seriously by the matter-of-fact individual who prides himself on believing only what he sees and distrusts suggestions which seem to contradict his own personal experience. It is therefore essential to realise that Einstein's theory stands or falls by observed facts: it aims at describing how things such as matter, time, and space do really behave. If, and when, any one can offer a simpler and more comprehensive account of the outdoor world than Einstein's theory is able to do, then Relativity will be superseded or modified. But at the present time it is contended that no other picture of the Universe, as adequate as Einstein's, exists. The story of scientific progress illustrates how indigestible new ideas are as a diet for plain, blunt men, but equally that those ideas which stand the test of time are easily assimilated by the plain, blunt men of a later date.
The Ptolemaic System.
Tradition suggests that Pythagoras (550 B.C.) was the first to teach that the Earth is a sphere, poised in space. This puzzled the man in the street in two respects: (i) How was the Earth supported? (ii) Why did not people or things on the other side of the Earth, being upside down, fall off?
Here, then, was one of the first of a series of shocks scientists have administered to common sense and will doubtless continue to administer as long as knowledge increases. But many centuries passed before the sphericity of the Earth was generally accepted, at any rate outside Greece; in fact it needed the circumnavigation of the world to drive it home as a matter of practical experience. Even to-day there are some who still maintain the Earth is flat (cf. Kipling's story, "The Village that voted the Earth was Flat," in A Diversity of Creatures), just as there are others who still try to square the circle. The Greek scientists, however, waived criticism aside and proceeded to calculate the circumference and diameter of the Earth. Aristotle (350 B.C.) states that the mathematicians of his time made the circumference 400,000 stadia (probably about 40,000 miles), but a far more accurate result was obtained by Eratosthenes (250 B.C.), who gave the circumference as the equivalent of 24,700 miles and the radius as 3925 miles, a degree of accuracy far higher than the rough methods he employed justify; it was in fact a lucky result. A later but less accurate measurement was made by Ptolemy (140 A.D.), whose treatise, largely astronomical, The Almagest, dominated scientific thought for the next fourteen centuries. The Ptolemaic System placed the Earth at the centre of the Universe, and regarded the Sun and the planets as moving round it in paths built up of circle and epicycles (i.e. circles rolling on circles); and although as time passed minor modifications were made to bring the theory into closer accord with observation, the general principle was accepted without question, till the time of Copernicus. It may be of interest to record that a contrary view had been put forward before Ptolemy's time. A certain Aristarchus of Samos (310-230 B.C.) maintained that the Sun was the centre of the world and that both the planets and the Earth moved round it. But this was far too advanced a doctrine not only for the common man but also for the scientists of his day; it offended their sense of propriety in that it degraded the Earth from the central to a subordinate position in Nature, and it outraged common sense to suppose that the Earth, which, as they said, any one could see and feel to be at rest, was really travelling through space more than a million miles a day. [Eratosthenes estimated the distance of the sun as 80,000,000 miles.] We are all now so accustomed to think of the Sun as the centre of the solar system that it is difficult to realise what a shock the normal man received when the doctrine was first seriously propounded.
The Copernican System.
The theory of Copernicus, De Revolutionibus orbium Coelestium, was published in 1543. It regarded the Sun as at rest at the centre of the world with the Earth and the other planets moving round it in circular orbits. The laborious work of Kepler (1571-1630), although showing that the orbits were not circular, gave powerful support to the heliocentric principle of Copernicus by establishing that the planets could be regarded as moving in ellipses with the Sun at one focus, and that the sizes of the orbits and the times and rates of description obeyed two simple quantitative laws, which later on assisted Newton to formulate his law of Universal Gravitation. The invention of the telescope in 1608 led Galileo (1564-1642) to discover Jupiter's moons, and this small-scale model of the solar system convinced him of the truth of the Copernican theory. After much hesitation and with considerable trepidation, which after-events fully justified, Galileo published in 1630 an account of his discoveries and beliefs. His thesis was not only a shock to the man in the street but, unhappily for Galileo, a shock also to the Church. In self-defence, he begged his opponents to come and look through his telescope, but neither the professors nor the ecclesiastics would do so. He was summoned to Rome, an old man in feeble health, tried by the Inquisition, and forced to "abjure, curse, and detest his errors and heresies." He died, a broken man and blind, near Florence in 1642. Einstein has good cause to be thankful that there is no Inquisition in power to-day.
Universal Gravitation.
The work of Copernicus, Kepler, Simon Marius, and Galileo was crowned by the publication of Newton's Principia in 1685. The notion that bodies fell to the earth owing to some form of attraction exerted by the earth did not originate with Newton. His genius showed itself in extending this idea to the whole Universe, formulating his result in a single law, and verifying it by an examination of the motion of the planets, comets, the Earth, and the Moon. Newton's troubles lay with his brother-scientists, not with ordinary folk, and many years of his life were embittered by the professional controversies which the Principia evoked. It has been said that there are comparatively few scientists who can, at the present time, really understand the mathematical work which is the scaffolding of Einstein's theory, but there were relatively far fewer in Newton's day who could appreciate the reasoning of thePrincipia; and of course a long time elapsed before Newton's ideas became part of the equipment of the ordinary educated man, as they are to-day.
The Mechanics of Galileo and Newton.
It is necessary to state, however briefly, the fundamental principles upon which Newton, using the observations of Kepler and the ideas of Galileo, based his system of celestial mechanics. He followed Galileo in saying that the nature of a body is such that, if at rest it will remain at rest, and if in motion it will continue to move uniformly in a straight line, unless there is some external cause operating to produce a change. This is the Principle of Inertia, and to the external cause he gives the name of Force and therefrom develops his idea of Mass.
For the working out of his mechanics, he postulates:
(i) The notion of absolute time: time flows uniformly and without reference to anything else.
(ii) The notion of absolute space: a fixed standard of reference, immutable and immovable, enabling the position or motion of any object in the Universe to be determined. The Earth is not at rest, the Sun may not be at rest, but there is, so Newton says, something existing in the Universe which will act as a fixed frame for defining absolute position and absolute motion.
The normal man neither had nor has any difficulty in assenting to these suppositions; in fact they seem so natural that he is shocked when asked to question them. The idea of universal gravitation was, on the other hand, far more perplexing. It appeared to involve the notion of "action at a distance," whereas everyday experience pointed to the belief that the action of one body on another was either caused by direct contact or some concrete connecting agency. Newton himself appears to have thought that further explanation was needed. Today it is an idea that the normal man accepts without protest; for him time and tradition have, as always, acted as the necessary shock-absorbers; but none the less since Newton's time a succession of scientists has attempted by a variety of physical theories to bridge the gulf.
Measuring Instruments.
The human senses, unaided by mechanical assistance, are not adapted for making accurate measurements either of time or space.
TIME.—Our sense of duration for periods of any length depends mainly on whether our occupation is interesting or tedious: our estimate of short periods of time is often ludicrously inaccurate. This may be illustrated by testing the ability of any one to judge the length of a minute, ruling out, of course, the assistance that counting, either aloud or mentally, affords.
The use of the sun-dial, which treats the Sun as a clock, is known to date back to 1500 B.C. in Egypt, and according to tradition it was introduced into Greece from Babylon by Anaximander in the sixth century B.C.; about this time also hour-glasses and water-clocks began to be made. Clocks depending on trains of wheels driven by a falling weight were used by the Romans in the sixth century A.D. The pendulum clock was invented by Huygens in 1673, ninety years after Galileo had discovered the isochronous property of a pendulum: Galileo himself used for his experimental work a form of waterclock which gave surprisingly good results. The first ship's chronometer of a reliable nature was constructed by a Yorkshireman, John Harrison, in 1761. This was an invention of immense practical importance, as at that time ships at sea could only determine their longitude by means of a clock. Under present conditions it is of less importance, because Greenwich mean time is sent out by wireless at noon each day.
SPACE.—Any one who visits Oxford should go and see the Evans Collection of ancient scientific apparatus at the old Ashmolean. The delicacy and accuracy of workmanship of the rulers, compasses, and astrolabes (i.e. instruments for measuring angles fixing the positions of the stars), some of which date back to very early times, can only be appreciated by seeing them. Chaucer wrote a treatise on the use of the astrolabe to make sure that his son should be properly instructed in its theory when he went to Oxford. The principle of the vernier, applied first to circular arcs, was discovered by a Portuguese named Nunes in 1542, and rediscovered by the Frenchman Vernier in 1631.
Artificial Extension of the Senses.
No great advance was possible in astronomy until the invention of the telescope in 1608. Naturally such an instrument at once aroused widespread attention, and within a few years telescopes were being used by scientists all over Europe. It not only increased the range of observation in astronomy, but it raised the degree of accuracy of measurement to a level far higher than had previously been possible. Modern discoveries are largely the fruit of two other inventions: (i) dry-plate photography, and especially its application to astronomy; (ii) the spectroscope and spectrum analysis. It is outside the province of this book to give any account of the amazing variety of application and the remarkable refinements of measurement yielded by these methods of research. The important point to observe is that the increase of knowledge of the Universe is due entirely to the successive aids that inventions have given in supplementing the powers of naked eyes and naked hands. Without such assistance, our knowledge of the structure of the world would remain very restricted. Without a telescope, we can only see details of things in our immediate neighbourhood, and that only to a limited extent; to fill in further detail, a microscope is necessary. Our ears only enable us to hear notes of limited pitch; our eyes are sensitive to a range of colour far more limited than the range of the photographic plate; if events happen too rapidly, our brains receive merely a blurred impression which it needs a slow-motion film to disentangle.
Common Sense.
Most of our outlook on life is coloured by the impressions we receive through senses, unaided by any artificial assistance. What we call a common-sense view of life is largely based on an acquaintance with things, confined in size between fairly narrow limits, restricted to small ranges of temperature and pressure, moving at low speeds and for short periods of time. It is not unreasonable to say that this gives us as true a view of the Universe as (say) a tourist could obtain of the interior of Westminster Abbey by looking through the keyhole of a side entrance. Successive inventions have enabled scientists to enlarge the keyhole, and perhaps at some future date will even throw open the door. If Science by its study of things very small and very large, very near and very distant, of temperatures very great, of velocities very high, is driven to conclusions which seem to violate our common-sense attitude, our keyhole notion of the Universe, it seems reasonable to treat it merely as one more shock in the succession which the man in the street has encountered and eventually absorbed. Nature is a conjurer for supermen. Generations of scientists have attempted to penetrate her secrets. Bit by bit the disguise is being torn away, but each new discovery seems only to open out fresh avenues demanding further exploration. Nature is a true woman, who will have the last word. Scientists of every age may well echo Newton's account of his own life's work: "I do not know what I may appear to the world, but to myself I seem to have been only like a boy playing on the seashore, and diverting myself in now and then finding a smoother pebble or a prettier shell than ordinary, whilst the great ocean of truth lay all undiscovered before me."
EXERCISE I
1. Why do you sometimes see on a cinematograph the wheels of a car rotating in a clockwise direction while the car itself is moving to the left?
2. A stone is tied to the end of a string and is whirled round in a circle horizontally. In what direction does the stone move when the string breaks?
3. If you assume that the earth describes a circle of radius 93,000,000 miles 365¼ in days, how many miles does it move in a second?
4. Galileo found it easier to show that the acceleration of a falling body is constant by considering motion down a slope instead of vertically. How could a cinematograph operator improve on Galileo's treatment?
5. If you drop a stone and a feather at the same moment, do they hit the ground simultaneously? Is your answer consistent with the statement that the acceleration due to gravity is the same for all bodies at the same place, regardless of their weights?
6. A camera photographs a 100 yards race for a cinema film, taking 150 exposures per second. They are reproduced on the screen at the rate of 15 per second; how long, roughly, will the race appear to the audience to last?
7. Describe a vernier, and show how one can be made to read correct to 1/100th inch.
8. Taking the length of the Equator as 25,000 miles, find the error in miles of the longitude of a position on the Equator, calculated from the record of a chronometer, if there is an error of one minute in the time.
9. Eratosthenes found that the sun was in the zenith at Syene when it was 7° 12' south of the zenith at Alexandria, which was known to be 5000 stadia north of Syene. What expression for the radius of the Earth can be deduced from these data?
(Continues...)
Excerpted from READABLE RELATIVITY by CLEMENT V. DURELL. Copyright © 2014 Dover Publications, Inc.. Excerpted by permission of Dover Publications, Inc..
All rights reserved. No part of this excerpt may be reproduced or reprinted without permission in writing from the publisher.
Excerpts are provided by Dial-A-Book Inc. solely for the personal use of visitors to this web site.
Table of Contents
1. The Progress of Science2. Alice Through the Looking-Glass
3. The Velocity of Light
4. Clocks
5. Algebraic Relations
6. Separation of Events
7. The Fourth Dimension
8. Mass and Momentum
9. General Relativity
10. The Einstein Tests
Answers