5
1
9780128160794
Waste-to-Energy: Technologies and Project Implementation / Edition 3 available in Paperback, eBook
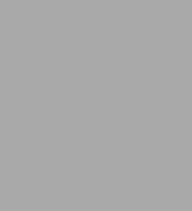
Waste-to-Energy: Technologies and Project Implementation / Edition 3
- ISBN-10:
- 0128160799
- ISBN-13:
- 9780128160794
- Pub. Date:
- 03/14/2019
- Publisher:
- Elsevier Science
- ISBN-10:
- 0128160799
- ISBN-13:
- 9780128160794
- Pub. Date:
- 03/14/2019
- Publisher:
- Elsevier Science
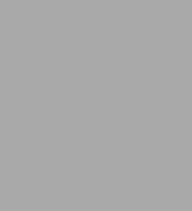
Waste-to-Energy: Technologies and Project Implementation / Edition 3
$160.0
Current price is , Original price is $160.0. You
160.0
In Stock
Product Details
ISBN-13: | 9780128160794 |
---|---|
Publisher: | Elsevier Science |
Publication date: | 03/14/2019 |
Edition description: | 3rd ed. |
Pages: | 228 |
Product dimensions: | 6.00(w) x 9.00(h) x (d) |
About the Author
What People are Saying About This
From the B&N Reads Blog